Introduction
In rice field, the occurrence of weeds has always some serious adverse impacts such as limiting the production of rice and increasing economic losses (Smith, 1983). The yield losses in rice due to weeds could be from 10 to 100% (Kim and Ha, 2005). Oerke and Dehne (2004) reported that worldwide yield losses in rice due to weeds was around 10% of the total rice yield. Acetolactate synthase (ALS)-inhibiting herbicides can effectively kill many weeds (Koschnick et al., 2007; Sprague et al., 1999). However, excessive and frequent use of ALS-inhibiting herbicides led to the evolution of herbicide resistant weeds (Hanson et al., 2013). High density of the ALS-inhibiting herbicide-resistant Schoenoplectus juncoides, a noxious weed in the paddy field, caused 40% loss of rice-yield. (Kuk et al., 2002; Park et al., 2005; 2006; Sada et al., 2013). Moreover, the occurrence area of herbicide-resistant S. juncoides in paddy field has increased from 7,032 ha in 2012 to 14,963 ha in 2018 in Chungcheongnam-do of Korea (Lee et al., 2012; Won et al., 2018). It is necessary to rapidly characterize whether the S. juncoides biotypes have evolved resistance to ALS-inhibiting herbicide so that the existing management strategies can be altered before the ALS-inhibiting herbicide resistant populations spread far and wide.
The whole-plant bioassay is a conventional method for detection of the ALS-inhibiting herbicide-resistance in weeds (Burgos, 2015; Guo et al., 2018; Joseph et al., 1990; Lee et al., 2018; Shim et al., 2020). In the whole plant bioassay method, the seedlings of the suspected ALS-inhibiting herbicide-resistant weed biotypes are grown from seed in greenhouse along with a known susceptible population and sprayed with the ALS-inhibiting herbicides each at different rates including the recommended rate. Then, the dry matters of the shoots of the suspect R biotypes are compared with the known susceptible population to determine the herbicide resistant populations. However, this method requires a long time, a lot of space, and a large amount of labour and money (Zhang et al., 2015). These limiting factors affect the detection of resistance in a large number of samples within a short period of time.
For a quick detection of ALS herbicide-resistance at a cheaper cost, the petri dishes method that is based on the physiology was developed (Abdurruhman et al., 2018; Burgos, 2015). The resistance in-season quick (RISQ) test, a petri dish analysis, can discriminate effectively between herbicides-resistant and -susceptible grass weeds and predict herbicide-efficacy prior to field application and thus allows for a reasonable choice of herbicide for effective control of weed (Davies et al., 2017; Kaundun et al., 2014). Kaundun et al. (2011) identified the herbicide resistant Lolium spp. by the Syngenta RISQ test (Kaundun et al., 2011) within 10 days. In addition, the cutting root test and cutting shoot test are doable by the farmers in a convenient and efficient way. Boutsalis et al. (2001) reported that identification of herbicide-resistant Alopecurus myosuroidess biotypes was possible in less than four weeks by cutting plants early in the season.
The objective of this study was to assess the feasibility of three rapid diagnosis methods including cutting root test, cutting shoot test, and RISQ test to identify rapidly ALS-inhibiting herbicide-resistant S. juncoides within the season.
Materials and Methods
Seed source and plant materials
Three populations (designated as ‘R’) of S. juncoides (GR, 217R, and WR biotypes) that were previously confirmed as resistance to ALS-inhibiting herbicides (data not shown), were collected from paddy fields of Chungcheongnam-do and Jeollabuk-do of Korea in April 2017. One herbicide-susceptible biotype (designated as ‘HS’) of S. juncoides (Jia et al., 2018) was collected from Chungcheongnam-do. After collection, plants of these four biotypes were grown in the greenhouse of Chungnam National University (36°37'02.72", 127°35'41.37") to propagate seeds. Seeds collected at the maturity of each biotype from the greenhouse were air-dried and stored in the refrigerator at 4℃ until used in the experiment.
Seeds of 217R, GR, WR, and HS biotypes were germinated at 28℃ and the seedlings were cultured under metal halide light to provide a 12-h photoperiod from 5 a.m. to 5 p.m. until the flowering stages.
Rapid bioassay for resistance confirmation
Roots of plants in the tiller stage were cut to retain 1 cm from the base of shoots after cleaning the whole plants by tap water. Then, the roots and shoot bases were dipped in the culture solution in a plastic bottle (Φ: 6.4 cm, H: 6 cm) and incubated with metal halide light to provide a 12-h photoperiod from 5 a.m. to 5 p.m. at 20/30℃ light/dark. The culture solution consisted of 1/20 Murashige and Skoog (MS) medium with flucetosulfuron and penoxsulam. The flucetosulfuron was applied at 4.89×10-4 to 25.60 mg a.i. L-1 to the 217R, GR and WR biotypes, and at 4.89×10-4 to 1.25×10-1 mg a.i. L-1 to the HS biotype. The penoxsulam was applied at 3.66×10-4 to 24.00 mg a.i. L-1 to the WR biotypes, and at 3.66×10-4 to 9.36×10-2 mg a.i. L-1 to the 217R, GR and HS biotypes. Culture solution without herbicide (untreated control) was also included for comparison. The length of root regrowth for each plant was measured two weeks after dipping in culture solution. The experiment was conducted in the randomized complete block design with three replications.
Shoots were treated with herbicide after cutting those 5 cm above the ground, and incubated in growth chamber for three weeks. The herbicide consisted of penoxsulam and flucetosulfuron at concentrations of 0, 0.75, 7.5, 15, 150, and 300 g a.i. ha-1, respectively. The experiment was conducted in a completely randomized design with three replicates. The maximum shoot length was measured three weeks after herbicide treatment.
The powdery agarose (G1002, Genocell, Jeonju, Korea) was added to 1/20 MS medium at 0.8% wt/V, melted in a microwave and then cooled to around 45 to 50℃ before adding to the penoxsulam and flucetosulfuron at concentration of 0, 1, and 100 µM. The mixture was poured immediately to a depth of 1.5 cm in 6 cm diameter petri dishes. The petri dishes with solidified agarose were placed in a refrigerator at 4℃ for 24-h prior to transplanting. Seedlings were horizontally transplanted on top of the agar senven days after germination. The roots were gently pushed into the agar using tweezers, ensuring the contact with the agar. The petri dishes were covered with a membrane of stretched Parafilm “M” and placed in growth chamber at 20/30℃ dark/light. The number of root was counted two weeks after transplanting. A seedling was identified as resistance if it grows new healthy roots. The experiment was conducted with three replications.
Statistical analysis
In the cut root test, the data were expressed as the percentages of untreated control estimates. A non-linear regression equation (1) below was used in SigmaPlot 12.5 (Systat Software, San Jose, CA, USA) to explain the relationship between the root length and herbicide efficacy.
(1)
where y is root length; x is the dose rates of ALS-inhibiting herbicides; a is the minimum value; d is the maximum value; c is GR50 (the herbicide dose required for 50% biomass reduction compared with the untreated); b is proportional to the slopes around the dose of GR50. Other experimental data (such as cut shoot and RISQ test) were analyzed by ANOVA (SPSS 21.0, Chicago, USA) with Tukey’s method at P=0.05.
Results
Cutting-root test
Cutting root method was used for confirming the ALS-inhibiting herbicide-resistant S. juncoides biotypes until the flowering stage. The WR biotype was highly resistant to the flucetosulfuron and penoxsulam (Fig. 1). GR50 values of the WR biotype to flucetosulfuron and penoxsulam were 4,999 and 2,370 µg a.i. L-1, that were 1,852 and 912-fold more resistant than the HS biotype, respectively (Table 1). The 217R and GR biotypes were highly resistant to the flucetosulfuron but were not resistant to penoxsulam (Fig. 1). The level of resistance for the 217R and GR biotypes was 100- and 34-fold more resistant than the HS biotype to flucetosulfuron, respectively. The GR50 values of the GR and 217R biotypes to penoxsulam were similar to that of HS biotype (Table 1).
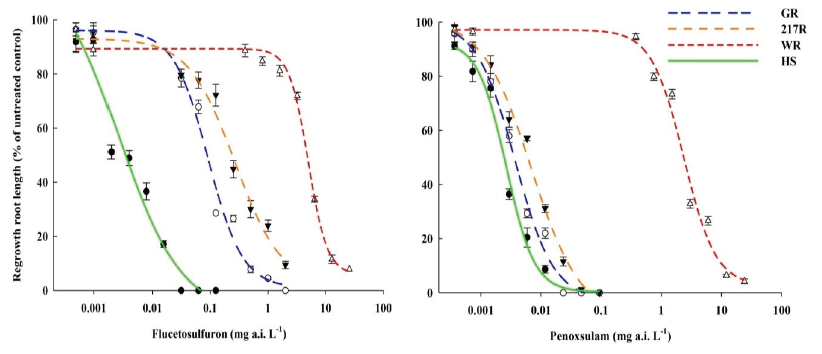
Fig. 1. Dose-response for regrowth root length of the susceptible biotype HS (●) and acetolactate synthase (ALS)-inhibitor-resistant biotypes GR, 217R, and WR (○, ▼, and △) of Schoenoplectus juncoides with a range concentration of flucetosulfuron and penoxsulam. Data were analysed using the log-logistic equation. Vertical bars represent 95% standard error of the mean.
Cutting-shoot test
The cutting-shoot test was used to identify the herbicide resistant S. juncoides in-season. With increases in the concentration of the flucetosulfuron and penoxsulam, the regenerative lengths of shoots of GR, 217R and WR biotypes decreased (Fig. 2). The shoot of the GR, 217R, and WR regrew 3.9, 4.2, and 11.4 cm at 300 g a.i. ha-1 concentration of the flucetosulfuron, which were 13.7, 18.3, and 57.6% of the shoot in the untreated plants, respectively. The resistance-level of the WR biotype was obviously greater than the GR and 217R biotypes. The shoot of HS biotype had no regrowth at 150 g a.i. ha-1 concentration of the flucetosulfuron. Additionally, the shoots of the GR, 217R, and the HS biotypes regrew less than 5 cm at 15 g a.i. ha-1 concentration of the penoxsulam. The regrowth of shoot of WR biotype was hardly inhibited at 15 g a.i. ha-1 concentration of the penoxsulam (Fig. 2). With the increase in the concentration of the penoxsulam to 300 g a.i. ha-1, the regrowth of shoot of WR biotype was significantly inhibited.
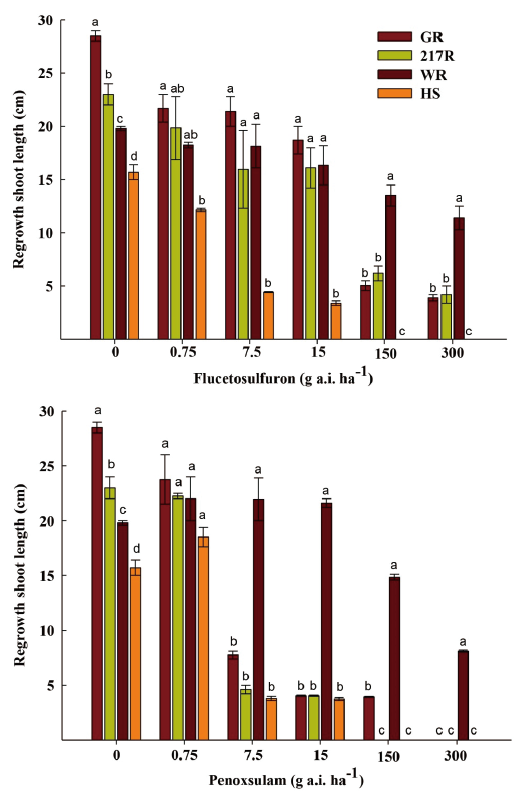
Fig. 2. The regrowth shoot length of Schoenoplectus juncoides three weeks after flucetosulfuron and penoxsulam treatment at various concentrations of 0.75, 7.5, 15, 150 and 300 g a.i. ha-1. a-d: Data followed by different letters with in the same rate of herbicide in same varieties indicate significant difference at the 0.05 level by Tukey’s test. Vertical bars correspond to standard error.
RISQ test
The result of RISQ test demonstrated that the GR, 217R and WR biotypes could survive after flucetosulfuron and penoxsulam treatment. The new roots of the GR, 217R and WR biotypes could regrow at 100 µM concentration of flucetosulfuron and penoxsulam (Fig. 3). Especially, the number of root for WR biotype (approximately 4.5 roots per plant) was significantly more than GR and 217R biotypes at 1 µM concentration of flucetosulfuron and penoxsulam treatment. The herbicide susceptible biotypes (HS) had no root growth at 1 µM concentration of flucetosulfuron and penoxsulam. In contrast, the HS biotype grew new roots without addition of any herbicides in the untreated petri dish.
Discussion
In this study, the cutting root test, cutting shoot test, and RISQ test were conducted to investigate rapidly detecting the ALS-inhibiting herbicide resistant biotypes of S. juncoides during the cropping season. The R biotypes (GR, 217R, and WR) were used as experimental materials for verification of three methods of rapid bioassays in Korea. In cutting roots test, the R biotypes (GR, 217R, and WR) could grow new roots after flucetosulfuron and/or penoxsulam treatment, while the HS biotype could not regrow at all (Fig. 2). These results in the cutting roots test were consistent with the dose response results in the whole-plant bioassay method (data not shown). Similarly, Hamamura et al. (2003) suggested that the roots of susceptible S. juncoides could be completely inhibited after bensulfuron-methyl treatment whereas no effect in SU-herbicide resistant biotypes was observed. In addition, the shoots of the GR, 217R, and WR biotypes could regrow after flucetosulfuron and penoxsulam treatment, while the HS biotype had no regrowth shoot. The cutting shoots test also could be used for identifying the ALS inhibiting herbicide resistant S. juncoides until flowering stage. However, the cutting shoots test takes a longer time than the cutting roots test. Therefore, the cutting root test is more suitable for famers to detect herbicide resistant S. juncoides individuals.
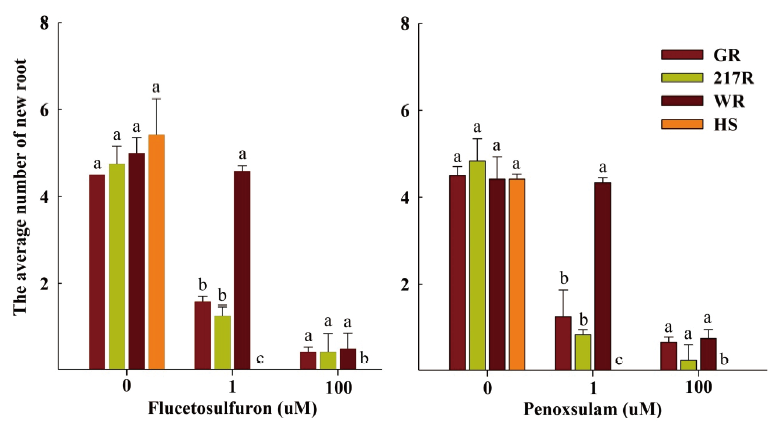
Fig. 4. The average number of new root for both herbicide resistant (R) and susceptible (S) populations of Schoenoplectus juncoides in agar medium containing flucetosulfuron and penoxsulam. a-c: Data followed by different letters with in the same rate of herbicide in same varieties indicate significant difference at the 0.05 level by Tukey’s test. Vertical bars correspond to standard error.
The RISQ test was developed firstly by Syngenta to detect herbicide resistant grass weeds. Result showed that the herbicide resistant Avena fatua, A. myosuroides, Setaria viridis, Apera spica-venti, and Phalaris paradoxa could survive after pinoxaden treatment, while susceptible one was unable to survive (Kaundun et al., 2011). In addition, Abdurruhman et al. (2018) detected acetyl-CoA carboxylase (ACCase) inhibitors resistant Avena sterilis based on the Petri dish analysis containing agar medium. The germination rate of the ACCase-inhibitor-resistant A. sterilis was more than the susceptible biotype after pinoxaden and clodinafop treatment. However, the previous research focused only on detecting the ACCase-inhibiting herbicide resistant grass weeds using the RISQ test. In our study, the GR, 217R and WR biotypes could survive after flucetosulfuron and penoxsulam treatment in RISQ test (Fig. 4). The modified RISQ test can be applied for rapid diagnosis of the herbicide resistant S. juncoides. However, the RISQ test is also inadequate because it requires a lot of monetary input and not as easy for the farmers to use as the cutting root test.
To-date, various methods have been described for detecting herbicide resistant weeds including molecular basis analysis, enzyme basis analysis, and chlorophyll fluorescence imaging (CFI) analysis. Polymerase chain reaction amplification of specific alleles (PASA), a molecular basis analysis, was useful to identify the ALS inhibitor resistant Amaranthus spp. and to serve as a monitoring tool (Wagner et al., 2002). The high-throughput ARMS/Scorpion real-time quantitative PCR (Q-PCR) assay provided effective and accurate detection of the ACCase inhibitor resistant Lolium spp. by measuring the relative frequencies of mutant L1781 alleles and I1781 wild-type alleles (Kaundun et al., 2006). In addition, the difference of the accumulation and activity of enzyme could be used for identification of herbicide-resistant weeds (Mao et al., 2016; Marshall and Moss, 2008; Reade and Cobb, 2002; Shaner et al., 2005; Yang et al., 2007). The CFI analysis, a reliable method, was conducted for identification herbicide-resistant Alopecurus spp. and Digitaria sanquinalis by detecting the photosynthetic activity after herbicide treatment (Chen et al., 2015; Hamouzová et al., 2011; Menegat and Gerhards, 2014; Menegat et al., 2011). Wang et al. (2016) demonstrated that the maximum photosynthesis system II quantum yield of susceptible A. myosuroides decreased significantly 3 days after ALS and ACCase inhibitors treatment, while there was no influence on the herbicide-resistant biotypes. These methods are potent, accurate, fast and highly repeatable, as well as the demonstrating the resistance-mechanism. However, these methods are only conducted in the laboratory and it requires a lot of monetary input and technical knowledge of the sophisticated equipment. By contrast, the rapid diagnosis methods including the RISQ test, cutting shoot and root test need less costs of labour and can easily identify the herbicide-resistant S. juncoides at seedling and tillering stages for avoiding the spread of seeds (Park et al., 2003).
Conclusions
Overall, in the face of occurrence of herbicide-resistant S. juncoides, the efficiency of identifying ALS-inhibiting herbicide-resistant S. juncoides at early stages of weeds and early in the season should be enhanced as much as possible. The rapid diagnosis of the ALS-inhibiting herbicide-resistant S. juncoides conducted by cutting root, cutting shoot test, and RISQ test in this study found that three methods can be applied for rapid diagnosis the ALS-inhibiting herbicide-resistant S. juncoides. Especially, the cutting root test is easier to apply in paddy fields for the in-season test of resistance to ALS-inhibitors than the other two methods. The famers can rely on the results of the present study to rapidly diagnose ALS-inhibiting herbicide-resistant S. juncoides and to select appropriate and effective management strategy at reduced costs of labour and herbicides in paddy field.
Acknowledgement
This work was carried out with the support of "Cooperative Research Program for Agriculture Science & Technology Development (Project No. PJ015723)" funded by Rural Development Administration, Republic of Korea.
Authors Information
Jia WeiQiang, http://orcid.org/0000-0002-1654-3638
Sug-Won Roh, Rural Development Administration Food Crop Industry Technology Service Division, Researcher
Jae Eun Song, Chungnam National University, Researcher
Aung Bo Bo, http://orcid.org/0000-0001-7579-3429
Kwang Min Cho, Daeseung Biofarm Research Center, Researcher
Kee Woong Park, http://orcid.org/0000-0003-0053-9543
Jeung Joo Lee, Gyeongsang National University, Professor