서언
잡초는 농경지에서 작물 생산량 감소의 원인이 되는 주요한 생물 인자이며, 제초제는 잡초를 방제하기 위한 가장 효과적이고 실질적인 수단이다. 그러나, 1940년대부터 유기 합성 제초제가 사용되기 시작한 이래 전 세계적으로 제초제 저항성 잡초의 발생이 지속적으로 증가하고 있는 실정이다. 오늘날 제초제 저항성은 전 세계적으로 273개 잡초종에서 보고되었으며, 그 수도 지속적으로 증가하고 있다(HRAC, 2024). 현재 전 세계적으로 식물의 다양한 생리 과정을 저해하는 제초제들이 잡초 방제를 위하여 사용되고 있다(Table 1). 또한, 저항성으로 알려진 잡초 종들은 작용 기구가 알려진 모든 주요 제초제에서 출현하고 있다(HRAC, 2024). 저항성은 제초제의 선발압에 대한 잡초 개체군의 진화적 적응의 결과이며(Neve et al., 2009), 그 특성상 target-site resistance (TSR)과 non-target-site resistance (NTSR)으로 구분할 수 있다(Beckie and Tardif, 2012; Délye, 2013).
우리나라의 논에서도 과거에 비해 근래에 이르러 제초제 저항성 잡초의 발생은 현저하게 증가하고 있는 실정이다(Table 2 및 3). 우리나라의 논에서 선택성 제초제를 사용하기 시작한 것은 1970년대초이며 butachlor를 비롯한 일년생 잡초의 방제를 위한 제초제들이 사용되기 시작하였다. 1990년대 초부터는 acetolactate synthase (ALS, 또는 acetohydroxyacid synthase; AHAS) 저해제인 sulfonylurea (SU)계 제초제가 사용되기 시작하였으며, 새로운 화본과 잡초 방제제인 acetylCoA carboxylase (ACCase) 저해 제초제들도 등장하여 ALS 저해 제초제들과 합제 형태로 사용되기 시작하였다(Park et al., 2005). 초기에 사용된 일년생 잡초 방제용 제초제들은 약효지속기간이 짧고, 여러 부위를 가해하기 때문에 저항성으로 변할 확률이 낮다. 그러나, ALS 및 ACCase 저해 제초제들은 선택성, 지속성 및 제초 활성이 높기 때문에 광엽 및 화본과 잡초에서 저항성의 발생이 증가하게 되었다(Park et al., 2011). 우리나라의 논에서 SU계 제초제에 대한 저항성은 1999년 최초로 물옥잠에서 보고되었으며(Park et al., 1999), 2009년 이후에는 화본과 잡초 방제용인 ACCase 저해 제초제에 대한 저항성 피의 출현이 보고되었다(Im, 2009; Lim et al., 2009; Park et al., 2010).
현재 우리나라의 논에서는 ALS 및 ACCase 저해제에 대한 저항성 잡초 종들의 종류와 발생 면적이 현저히 증가하고 있으나(Table 2 및 3), 저항성 기구에 관한 종합적인 연구는 부족한 실정이다. 따라서 본 리뷰는 제초제 저항성의 두가지 카테고리인 TSR 및 NTSR과 관련된 특성을 이해하고, 우리나라의 논에서 발생한 저항성 잡초와 어떤 관련성이 있는지를 파악하기 위하여 작성되었다.
제초제 저항성의 구성 요소
제초제는 (1)침투, (2)작용 부위로의 이행, (3) target 단백질 부위에 축적, (4) target 단백질에 결합 및 (5) 중요한 대사 경로나 구조적 기능을 방해하는 단계로 작용한다(Délye et al., 2013). 마지막의 (5) 단계는 세포 구성 요소를 손상시키고 식물의 죽음을 가속화하는 활성 산소종의 생성과 같은 부수적인 저해 효과를 동반할 수 있다(Fig. 1). 이와 같은 제초제의 살초작용에 대해 저항성 잡초는 TSR 이나 NTSR 메커니즘에 의해 피해를 극복하고 생장할 수 있다(Beckie and Tardif, 2012; Délye, 2013).
Fig. 1
The action of herbicides ((1), (2), (3), (4), (5), top) following their application and the resistance mechanisms identified in weeds that correspond to each action step ((A), (B), (C), (D), (E), (F), bottom). After application, (1) herbicide molecules penetrate the plant, (2) are translocated to the location of the target protein (here, the chloroplast of meristem cells), (3) accumulate at the location of the target protein, and (4) bind to the target protein, thereby (5) disrupting biosynthesis pathways or vital cell structures, and/or generating cytotoxic molecules (e.g., active oxygen that damage cells and ultimately causes plant death). Multiple mechanisms of resistance interfering with the herbicide action steps have evolved in weeds. Nontarget-site resistance (NTSR) mechanisms include (A) reduction in herbicide penetration due to alterations in cuticle properties and/or plant habit, (B) altered translocation of the herbicide away from the target protein, (C) enhanced degradation (metabolism) of the herbicide, or (D) enhanced neutralization of cytotoxic molecules generated by herbicide action (illustrated: neutralization of active oxygen by peroxidases). Targetsite resistance (TSR) mechanisms include (E) regulatory mutations causing target protein overproduction that compensates for the herbicide inhibitory action, and/or (F) structural mutations that modify the 3D structure and electrochemical properties of the target protein. Structural mutations can have no, moderate, or strong negative effects on the stability of herbicide binding to the target protein, which result in (F-a) no, (F-b) moderate or (F-c) marked reduction in herbicide sensitivity at the protein level, respectively; or can (F-d) increase the stability of herbicide binding to the target protein (Délye et al., 2013).
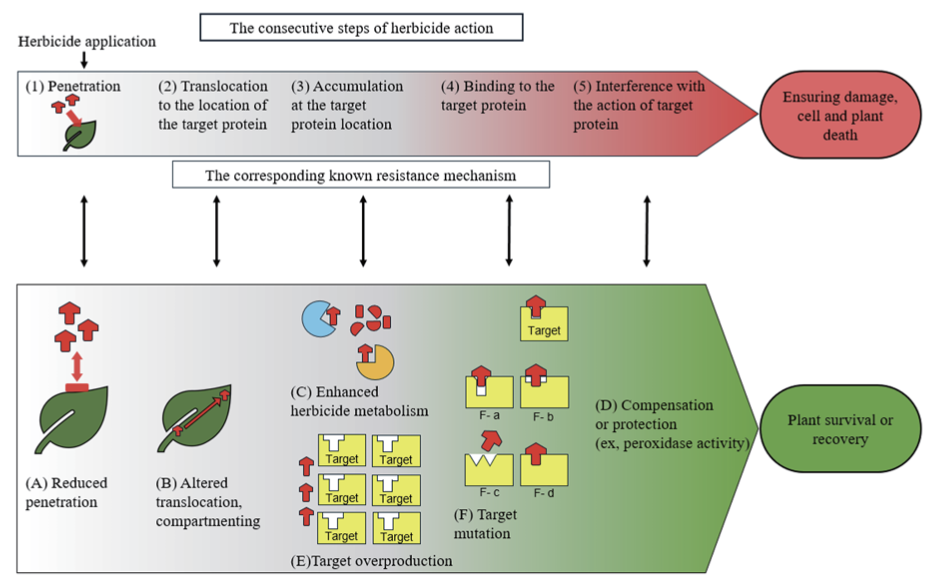
Target-site resistance (TSR)
제초제 저항성의 첫 번째 카테고리인 TSR은 제초제 target 단백질을 코딩하는 유전자의 돌연변이에 의해 아미노산이 치환되어 제초제 분자와의 친화력이 감소하거나(Fig. 1-Fa, b 및 c), target 단백질의 과잉 생산에 의해(Fig. 1-E) 일어날 수 있다(Avila-Garcia et al., 2012; Beckie and Tardif, 2012; Shaner, et al., 2012). TSR은 HRAC 1 (ACCase inhibitor), HRAC 2 (ALS 또는 AHAS inhibitor) 및 HRAC 5, 6 (photosystem Ⅱ inhibitor)에 속한 제초제들에 대해 광범위하게 존재한다(Table 1). 일반적으로 잡초에 저항성을 부여할 수 있는 다양한 치환체들이 target 단백질 유전자의 중요 코돈에서 나타날 수 있는데, 그룹 B 제초제의 target 단백질인 ALS (또는 AHAS)의 경우 코돈 197에서 저항성을 부여하는 코돈의 치환이 12개나 확인되었다(Beckie and Tardif, 2012). 결합 부위에 대한 제초제의 친화성 감소 정도는 target 단백질의 구조적 변화 및 제초제의 종류에 따라 다르다. Target 단백질의 구조적 변화는 제초제의 친화도에 따라 서로 다른 정도의 저항성을 부여하나(Délye et al., 2005; Yu et al., 2007) (Fig. 1-Fa, b 및 c), 드물게는 친화성을 증가시켜 제초제에 대한 감수성을 증가시킬 수도 있다(Fig. 1-Fd).
Non-target-site resistance (NTSR)
제초제 저항성의 두번째 카테고리인 NTSR은 TSR에 속하지 않는 제초제의 흡수, target 단백질로의 이행 및 축적(Fig. 1-A, B 및 C)의 단계 중 적어도 하나를 저해하거나, 제초제의 유해 작용을 보상하는 메커니즘(ex, peroxidase activity, Fig. 1-D)에 의해 유발된다. 제초제의 흡수 감소에 의한 NTSR (Fig. 1-A)은 glyphosate (Vila-Aiub et al., 2012), ALS (White et al., 2002) 및 ACCase 억제제(De Prado et al., 2005)에 대한 저항성 식물 등에서 보고되었다. 제초제의 흡수 감소는 큐티클 층의 차이로 인해 일어날 수 있으나, 제초제의 제제는 침투를 최적화하도록 설계되었기 때문에(Zabkiewicz, 2000), 이 유형의 메커니즘은 식물체에 높은 수준의 저항성을 제공하기 어려울 것으로 예상된다. 한편, 제초제의 이행 감소(Fig. 1-B)도 glyphosate 및 paraquat 저항성 식물에서 보고되었다(Powles and Yu, 2010). 작용점 부위에서 제초제 축적의 감소는(Fig. 1-C)는 NTSR에서 가장 중요한 역할을 하는 것으로 알려져 있는데 (Yuan et al., 2007; Van Eerd et al., 2003), 이 작용과 관련된 여러 주요 효소 들이 알려져 있다(Table 4).
일반적으로 제초제 분해는 여러 유형의 효소가 작용하는 과정이며 기본적으로 세 단계로 나눌 수 있다. 제 1 단계에서는 제초제 분자가 친수성의 대사물로 변형되며, 제 2단계에서는 변형된 친수성 대사물이 식물 수용체 분자(예: glutathione 또는 sucrose)에 접합되고, 제 3단계에서는 추가적인 접합, 절단 또는 산화를 거친 대사산물이 액포 또는 세포벽으로 이동된다(Délye, 2013). NTSR은 제초제의 유해 작용으로부터 식물 세포를 보호하여 유발될 수도 있다(Fig. 1-D). ACCase 억제제와 같은 일부 제초제는 지방산 생합성을 억제하는 것 외에도 세포 구성 요소를 손상시키는 활성산소종을 방출하기도 한다(Cummins et al., 2009). ACCase 억제제에 대한 저항성 쥐꼬리뚝새풀(A. myosuroides)은 활성산소 소거효소인 peroxidase의 발현량을 증가시켜 산화적 손상으로부터 세포를 보호한다. 그 결과 저항성 쥐꼬리뚝새풀은 더 오랜 시간 동안 제초제를 분해할 수가 있다. 이 경우 NTSR의 직접적인 원인은 증가된 제초제 분해 보다 제초제에 의해 생성되는 활성산소로부터 세포를 보호하였기 때문이라고 할 수 있다(Cummins et al., 2009). 이와 유사하게 paraquat에 대한 잡초 종의 저항성도 산화적 손상으로부터 세포를 보호하는 효소 활성의 증가에 의해 비롯되는 것으로 알려졌다(Preston, 1994). NTSR은 TSR보다 광범위하고 일반적인 잡초의 적응 과정에 의해 생성된 것으로 보인다(Délye et al., 2013). NTSR의 가장 우려되는 측면은 예측 불가능한 교차 저항성으로, 특정의 작용 기구를 갖는 제초제에만 국한 되지 않고 다른 작용 기구를 가진 제초제들에 대해서도 교차저항성을 부여할 수 있다. 따라서 NTSR에 의해 획득된 잡초의 저항성은 TSR에 의한 것보다 더 큰 문제가 될 수 있으며, 그러한 저항성 잡초를 방제하기 위하여 재배 방법의 개선, 다른 계통의 제초제 사용 및 효과적인 혼합제의 사용 등과 같은 대책을 강구해야 할 필요가 있다(Park et al., 2005).
NTSR의 진화
잡초는 유전적으로 이질적인 단위의 집합으로 구성되어 있음으로(Menchari et al., 2007; Osuna et al., 2011), 개체 간 제초제에 대한 민감성의 차이가 집단내에 존재할 수 있다(Neve and Powles, 2005a, Neve and Powles, 2005b). 이질적인 유전적 특성을 지닌 잡초군에 반복적으로 동일 제초제를 살포하면 NTSR 메커니즘과 관련된 대립유전자는 제초제의 선발압에 의해 개체군 내에서 출현 빈도가 증가하고 축적되며(Jasieniuk et al., 1996), 생장 주기의 반복과 더불어 NTSR은 점진적으로 진화한다(Fig. 2). 그 결과 작물 재배 포장에서는 제초제에 대한 저항성 개체의 출현 빈도가 증가할 것이며, 저항성이 더 높은 잡초가 발생할 것으로 예상된다(Délye, 2013).
Fig. 2
Evolution of NTSR by ‘gene stacking’. Herbicide applications select for weed genotypes with a reduction in sensitivity to herbicides owing to different alleles (shown as different symbols within the ovals representing the genotypes). These genotypes reproduce. Some of their progeny plants have accumulated different parental alleles, each causing a reduction in herbicide sensitivity. These genotypes can be less sensitive than the parent plants in terms of resistance level and/or resistance spectrum. After several cycles of selection, genotypes emerge that have accumulated many alleles conferring a reduction in herbicide sensitivity. The limits to this accumulation are the number of different alleles present in the population, and the maximum number of alleles that can beaccumulated in a single plant without causing a severe reduction in fitness (Délye, 2013).
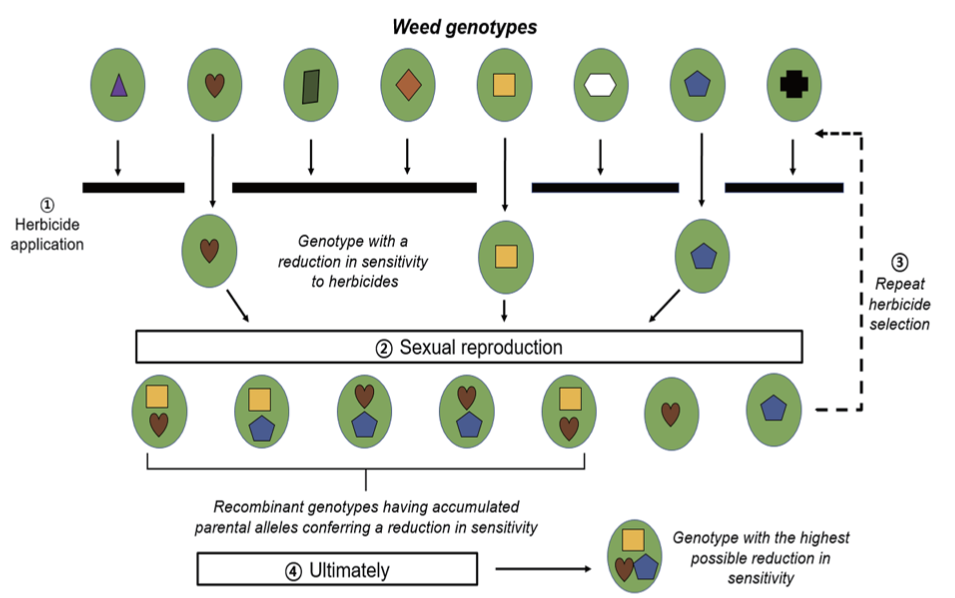
저항성 잡초의 생장 비용 및 이점
TSR 이나 NTSR에 의해 저항성이 유발된 잡초는 생장 적응을 위한 비용(a fitness cost)이 필요할 수 있다(Délye, 2013; Délye et al., 2013). 이러한 적응 비용을 다발성 발현 효과(pleiotropic effects)라 하며(Pavlicev and Wagner, 2012; Purrington, 2000), 이 효과는 식물 생리 및 발달 과정에도 영향을 미칠 수 있다(Vila-Aiub et al., 2009b). 그 예로 group A 제초제들에 대해 저항성을 발휘하는 ACCase 대립유전자는 biomass 및 종자 생산량에서 불리하게 작용할 수 있다(Menchari et al., 2008). 또한, group C 제초제들에 대해 TSR을 나타내는 식물의 경우 광합성 능력의 부족 때문에 종자 및 biomass의 생산, 경합 능력 등에 대해 불리한 다발성 발현 효과가 있는 것으로 밝혀졌다(Vila-Aiub et al., 2009b). 다른 제초제 그룹 들에서 다발성 발현 효과는 억제적이거나 촉진적일 수 있으며, TSR이나 NTSR의 종류에 따라서도 다르게 나타날 수 있다(Table 5). 저항성 대립유전자에 의한 다발성 발현 효과는 식물 종에 따라서도 달라질 수 있는데, AHAS 대립유전자 Trp-574-Leu과 연관된 다발성 발현 효과는 식물 종에 따라 biomass 및 경합 능력이 감소된 것부터 가시적인 효과가 없는 것에 이르기까지 다양하였다(Table 5). 또한, 다발성 발현 효과는 잡초 개체군의 생장에 유익할 수도 있는데, 강아지풀속(Setaria sp.)의 잡초에서 ACCase 대립유전자 Ile-1781-Leu의 경우 group A의 제초제들에 대해 TSR을 부여할 뿐만 아니라, 잡초의 biomass 생산량을 증가시키며(Wang et al., 2010), 이른 시기의 잡초 방제를 회피할 수 있도록 종자 발아를 지연시킬 수도 있다(Délye et al., 2013) (Table 5). 따라서 대립유전자의 이러한 다발성 발현 효과로 말미암아 제초제에 대한 저항성을 발휘하도록 진화된 잡초 개체군에서는 이 대립유전자가 널리 퍼져 있게 된다(Délye et al., 2010).
ALS 저해 제초제에 대한 저항성 잡초의 발생
2012년과 2017년에 전국적으로 실시된 ALS 저해 제초제 저항성 논 잡초에 관한 조사 결과, 저항성 논 잡초의 평균 발생률은 2012년에 전체 벼 재배면적의 22.1% 였으나, 2017년에는 63.2%로 현저하게 증가하였다(Table 2). 따라서 ALS 저해 제초제 저항성 논 잡초는 조사 년도가 경과할수록 보다 광범위한 면적에서 확산되고 있는 것으로 나타났다. 저항성 잡초종도 조사년도가 경과할수록 증가하였다(Table 3). 발생 면적이 10,000ha 이상 되는 저항성 잡초종은 2004년에는 물달개비, 알방동사니 및 물옥잠 등 3개 초종에 불과하였으나, 2008년에는 물달개비, 올챙이고랭이, 알방동사니 및 물옥잠 등 4개 초종, 2012년에는 물달개비, 논피, 올챙이고랭이, 미국외풀, 알방동사니, 여뀌바늘 및 밭뚝외풀 등 7개 초종, 2017년에는 물달개비, 논피, 돌피, 물피, 올챙이고랭이, 미국외풀, 알방동사니 및 여뀌바늘 등 8개 초종에 달하였다. 또한 2012년까지는 100,000 ha 이상 발생한 저항성 잡초가 없었으나, 2017년 조사에서는 저항성 물달개비, 논피를 비롯한 피속 잡초 및 올챙이고랭이가 각각 292,936 ha (38.2%), 180,753 ha (23.6%) 및 138,756 ha (18.1%)나 발생하였다. 2012년 대비 2017년도 조사에서 발생 면적이 10,000 ha 이상인 저항성 잡초의 초종별 증가율은 피속 잡초가 13.3배로 가장 높았고, 이외에도 미국외풀, 물달개비, 올챙이고랭이, 알방동사니 및 여뀌바늘이 각각 8.5배, 5.1배, 4.1배, 2.8배 및 1.3배의 순으로 증가하였다. 또한 저항성 벗풀의 발생 면적은 2012년 조사에서 불과 191 ha에 불과하였으나, 2017년 조사에서는 17.3배나 증가한 3,304 ha에 달하였다.
ALS 및 ACCase 저해 제초제에 대한 저항성 피의 발생
피속 잡초는 벼 재배 시 10 m2 당 7본만이 발생하여도 생산량의 50% 이상을 감소시킬 정도로 벼에 대한 경합력이 높은 것으로 알려져 있다(Moon et al., 2004). 제초제 저항성 피는 ALS 및 ACCase 저해제 계열의 제초제에 대한 저항성이 큰 문제가 되고 있다. Lee 등(2013)에 따르면 2008년에 ALS 저해 제초제에 대한 저항성 논피의 발생면적은 소규모(17 ha)였으나, 2012년에는 물달개비 및 올챙이고랭이에 이어 3위(13,581 ha)로 현저히 증가하였다. 2017년 조사에서는 SU계 제초제 저항성의 피속 잡초(논피, 돌피 및 물피)가 물달개비에 이어 두 번째로 넓은 면적(180,753 ha, 23.6%)에 발생하였다(Table 3). Im (2009) 및 Im 등(2008)은 각각 서산 간척지와 김제에서 ACCase 저해 제초제 저항성 논피 및 물피의 발생을 보고하였으며, Park 등(2010)은 익산, 김제 및 부안에서 채집된 논피에서 cyhalofop-butyl 및 penoxsulam에 대해 다양한 정도의 저항성이 있는 것으로 보고하였다. Lee 등(2017)의 보고에 따르면 ALS 저해제인 penoxsulam (30 g a.i. ha-1)과 ACCase 저해제인 cyhalofop-butyl (250 g a.i. ha-1)에 대해 전국의 피 발생지에서 채집된 논피(Echinochloa oryzicola) 및 돌피(Echinochloa crus-galli)의 저항성 정도를 검정한 결과, penoxsulam에 대해서 논피 44점(전체 수집종의 34.4%)과 돌피 46점(10.7%)이 종자 수확이 가능할 정도로 저항성을 나타냈었다(Table 6). 한편 cyhalofop-butyl에 대해서는 논피 25점(19.5%)에서 종자 수확이 가능하였으나, 돌피는 종자 수확시까지 생존하지 못하고 모두 고사하였다. 또한 논피 20점(15.6%)은 추천량의 penoxsulam 및 cyhalofop-butyl 처리에서도 생존하였다. 따라서 논피는 돌피보다 ACCase 및 ALS 저해제에 대해 높은 저항성을 나타내며, 그 중에는 이들 약제에 다중저항성을 갖는 biotype도 15% 이상 있는 것으로 나타났다. 이와 같은 결과로부터 전국에서 발생하고 있는 논피에서 ALS 또는 ACCase 저해 제초제에 대한 저항성 및 두 계통의 저해제들에 대한 다중저항성이 확산되고 있는 것으로 사료되었다.
여러 가지 ALS 저해 제초제 저항성 잡초 종의 GR50
ALS 저해 제초제에 대해 한국, 일본 및 중국에서 발생한 여러 저항성 잡초 종의 반응 정도를 조사한 결과, 각각의 제초제들에 대한 저항성 biotype의 GR50 비율은 잡초 및 제초제의 종류에 따라 매우 다른 것으로 나타났다(Table 7). 우리나라에서 발생한 저항성 물옥잠의 경우 ALS 저해 제초제들에 대한 감수성 대비 GR50의 비율은 ethoxysulfuron의 4.6배에서부터 pyrazosulfuron-ethyl의 63.5배까지(Park et al., 2002), 저항성 물달개비는 pyrazosulfuron-ethyl의 9배에서 imazosulfuron의 1,611배까지 차이가 났다(Kuk et al., 2004). 올챙이고랭이도 penoxsulam에 대한 감수성 biotype 대비 GR50 비율은 biotype TY238 및 HA135에서 경우 큰 차이가 없었으나, GS28은 127.3배로 매우 높게 나타났다(Shim et al., 2020). Flucetosulfuron에 대한 TY238 및 HA135의 GR50 비율은 각각 17.8 및 43.4배로 비교적 낮게 나타났으나, GS28의 비율은 269.5로 penoxsulam에서와 유사하게 매우 높게 나타났다. 따라서, GS28은 penoxsulam 및 flucetosulfuron에 대하여 매우 높은 저항성을 나타내나, TY238 및 HA135는 penoxsulam에 대해서는 감수성이고 flucetosulfuron에 대해서는 낮은 저항성을 갖는 것으로 사료되었다. 저항성 벗풀의 경우에도 각 나라별로 저항성 biotype의 GR50 비율은 달랐다. 한국에서 수집된 저항성 biotype의 경우 imazaquin, penoxsulam 및 cyclosulfamuron에 대한 GR50 비율은 각각 8, 13 및 25로 나타났다(Jia et al., 2021). 한편, 일본에서 수집된 저항성 벗풀은 bensulfuron-methyl에 대한 2개 저항성 biotype(R1 및 R2)의 GR50 비율이 모두 높았으나, pyrazosulfuron-ethyl에 대해서는 R1만 높은 저항성을 나타냈다(Iwakami et al., 2014). 또한, 중국에서 수집된 벗풀(R1 및 R2)은 bensulfuron-methyl에 대해서 모두 높은 저항성을 나타냈다(Wei et al., 2015). 이러한 결과로부터 여러 저항성 잡초는 분자 구조의 차이가 있는 ALS 저해 제초제의 종류에 따라 저항성이 다르게 나타나는 것을 알 수 있었다. 한편, 일본의 저항성 벗풀 R1 및 R2 biotype은 bensulfuron-methyl 및 pyrazosulfuron-ethyl에 대한 GR50 비율이 매우 다르기 때문에 저항성 기구도 서로 다를 것으로 예상되었다.
TSR 메커니즘에 의한 제초제 저항성 논잡초의 발생
우리나라에서 발생한 여러 종류의 ALS 저해 제초제 저항성 잡초의 als 유전자 염기서열을 조사한 결과, 물옥잠의 경우는 감수성 biotype의 168Thr (ACW), 189His (CAT) 및 247Asp (GAT)이 저항성 biotype에서 각각 Ser (TCW), Arg (CGY) 및 Glu (CAA)으로 전환되었다(Table 8). 이것은 als 유전자의 변이가 일어나는 주요 부위로 알려진 197Pro (CCT)이 아닌 다른 부위의 아미노산 치환에 의해서도 저항성이 유기될 수 있다는 것을 나타낸다. 물옥잠의 경우와는 다르게 물달개비는 197Pro (CCT)이 저항성에서 Ser (TCT, AB 243612)으로(Park et al., 2003), 벗풀은 197Pro (CCC)이 Leu (CTC, R)로 치환되었다(Jia et al., 2021). 또한, 일본에서 보고된 저항성 벗풀 R1은 197Pro (CCC)이 Ser (TCC)으로(Iwakami et al., 2014), 중국의 저항성 벗풀 R1 및 R2도 197Pro (CCC)이 Leu (CTC) 및 Ser (TCC)으로 치환되었다(Wei et al., 2015). 이러한 결과로부터 ALS 저해제에 대한 우리나라의 저항성 물옥잠, 물달개비 및 벗풀, 일본의 저항성 벗풀 R1, 중국의 저항성 벗풀 R1 및 R2 등의 저항성은 작용점 효소인 ALS의 197Pro (CCT 또는 CCC)이나 다른 부위의 치환에 의해 비롯되었으며, 그 저항성의 메커니즘은 TSR로 예상되었다. 또한, 올챙이고랭이 TY238 및 HA135도 ALS의 197Pro이 각각 Ser 및 Ala으로 치환되었으며 flucetosulfuron에 대해서 낮은 정도의 저항성을 나타냈으므로, TSR 메카니즘에 의해 저항성을 발휘한 것으로 사료되었다.
NTSR 메커니즘에 의한 제초제 저항성 논잡초의 발생
NTSR 메카니즘도 TSR 메카니즘과 더불어 제초제에 대한 저항성을 부여한다(Délye, 2013; Délye et al., 2013). P450은 식물체 내의 제초제 대사를 촉매하는 효소로 잡초에게 NTSR를 부여하는 주요 인자로 알려져 있으며(Barrett, 1995) (Table 4), 유기인계 살충제인 fenitrothion은 P450의 활성을 저해하는 자살 기질(suicide subatrate)로 작용한다(Berger and Sultatos, 1997). 앞서의 결과에서 올챙이고랭이 GS28은 als 유전자의 돌연변이가 일어나지 않았음에도 불구하고 ALS저해 제초제에 대해 강한 저항성을 나타내었다(Table 7, 8). 그런데 고성28에 P450의 저해제인 fenitrothion을 처리한 후 penoxsulam 및 flucetosulfuron을 살포한 결과, 제초제에 대한 저항성은 현저하게 감소하였다(Fig. 3). 이것은 fenitrothion에 의해 P450의 활성이 억제되어 제초제의 대사가 원활하게 진행되지 못하였기 때문으로 추정되었다. 한편, 일본에서 보고된 저항성 벗풀 R2의 경우에도 ALS 유전자의 돌연변이 없이 저항성을 나타냈음으로(Iwakami et al., 2014), 고성28과 유사하게 NTSR 메커니즘에 의해 저항성이 유래된 것으로 추정되었다. 따라서 이와 같은 사실로부터 제초제 저항성 잡초의 발생에는 TSR 뿐만 아니라 NTSR 메카니즘도 중요한 역할을 할 수 있는 것으로 사료되었다.
Fig. 3
Effects of fenitrothion and ALS inhibitors on the dry weight of Scirpus juncoides GS28 biotype. Data were analyzed using the log-logistic equation. Vertical bars represent 95% confidence interval of the mean at each rate. Fenitrothion was treated to the plants at 2,000 g a.i. ha-1 24 hours before herbicide treatment (Shim et al., 2020).
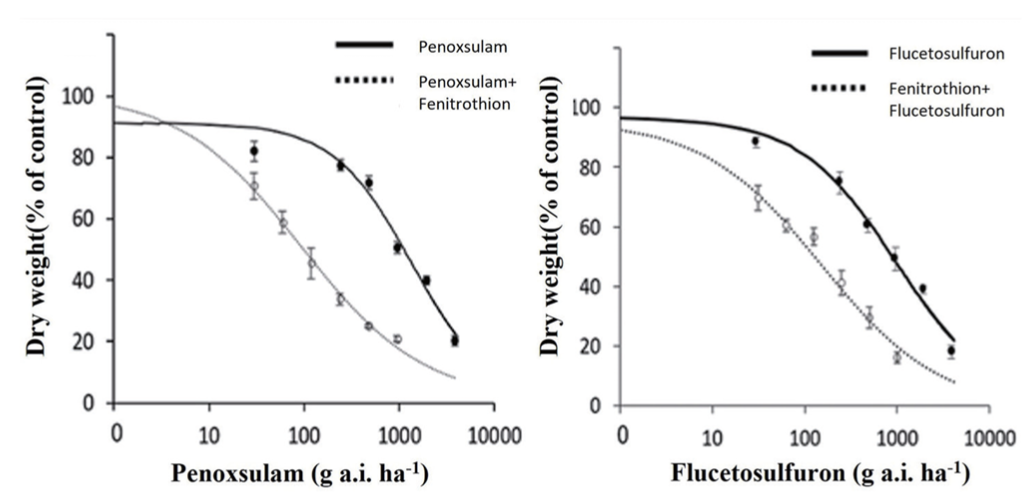
제초제 저항성 논 잡초의 생장 적합도(fitness)
우리나라의 경우 논에서 제초제 저항성 잡초의 종류 및 발생 면적은 근래에 이르러 뚜렷하게 증가하고 있다(Table 2 및 3). 그러나 제초제 저항성 잡초의 생장 적합도에 관한 연구는 아직 미미한 실정이다. 저항성 및 감수성 biotypes들에서 생장 적합도의 차이는 진화에 영향을 미치는 주요 요인이다(Warwick and Lynn, 1994). 제초제 선발압이 없는 경우에 저항성 biotype이 감수성 biotype보다 생장에 더 적합하다면 저항성 biotype은 개체군에서 우점할 것이다. Jia 등(2021)에 따르면 als 유전자의 Pro-197-Leu 돌연변이가 있는 저항성 벗풀은 감수성 biotype에 비해 종자 발아율이 높고 유식물의 신장 생장이 촉진되었으므로(Table 9), 생장적합도에서 유리하며 종자가 논에 전파된다면 우점종이 될 수 있을 것으로 예상되었다. 이와는 반대로 괴경에서 유래한 저항성 벗풀은 유식물의 생장이 감수성 벗풀보다 현저하게 억제되었음으로(Table 9), 생장적합도에서 불리하며 제초제의 선발압이 없는 경우에 감수성 biotype이 우세종이 될 것으로 예상되었다. 한편, 올챙이고랭이의 경우도 NTSR 변이를 갖을 것으로 예상되는 저항성 biotype(GS28)의 종자 발아율이 감수성 biotype 보다 더 높았으므로 생장 적합도도 더 높으며, 그 종자가 논에 유입된다면 감수성보다 우세종이 될 것으로 예상되었다.
제초제 저항성의 발생기구 연구
우리나라에서 가장 최근인 2017년에 실시된 조사에서 ALS 저해형 제초제에 대한 저항성 논 잡초는 물달개비를 비롯해 12종 이상에 이르는 것으로 나타났다(Table 3). 또한 ALS 및 ACCase 저해제에 대해 저항성 및 교차저항성을 나타내는 피의 발생도 보고되었다(Im, 2009; Im et al., 2008; Park et al.,2010; Lee et al., 2017). 그러나, 현재까지 제초제 저항성 기구의 구명에 필수적인 target 단백질의 아미노산 서열 변이가 밝혀진 잡초는 물옥잠, 물달개비, 올챙고랭이 및 벗풀에 불과하다. 만약 저항성 잡초의 target 단백질에 변이가 일어났다면 그 저항성은 TSR에 의해, 변이가 일어나지 않았다면 NTSR에 의해 유래되었을 가능성이 크며, TSR 및 NTSR의 공동 작용에 의한 저항성 발현의 가능성도 있다. 따라서 제초제 저항성의 구명을 위해서는 더 많은 저항성 잡초종들에서 분자생물학적 연구를 통한 target 단백질의 돌연변이 여부를 밝히는 것이 필수적이며, TSR이 아닐 경우 후속적인 연구를 통해서 NTSR에 의한 저항성의 발현 여부도 밝힐 수 있을 것이다. 또한, 이러한 체계적인 연구를 통하여 제초제 저항성 잡초의 발생을 방지하고 기 발생된 저항성 잡초의 방제에도 이용 가능한 대책을 마련할 수 있을 것이다.
요약
일반적으로 제초제 저항성은 target-site resistance (TSR)와 non-target-site resistance (NTSR)로 구분할 수 있다. TSR 메카니즘은 제초제 target 단백질을 코딩하는 유전자의 돌연변이에 의해 해당 단백질과 제초제 분자와의 결합이 감소하거나, target 단백질의 과잉 생산에 의해 발생할 수 있다. NTSR은 TSR과 달리 제초제의 흡수, target 단백질 부위로의 이행 및 축적의 단계 중 적어도 하나를 저해하거나, 제초제의 유해 작용을 보상하는 메커니즘에 의해 유발된다. 또한, NTSR은 제초제의 반복 처리에 의해 저항성 관련의 대립유전자가 축적되어 진화하며, 그 결과 저항성은 더 높아진다. 이 중에서 cytochromes P450을 비롯한 여러 효소들은 제초제의 분해 및 저분자 화합물과의 포합 반응을 통하여 작용점 부위에서 축적되는 제초제의 양을 감소시키거나 제초제의 유해 작용으로부터 세포를 보호하므로, NTSR에서 가장 중요한 역할을 수행한다고 볼 수 있다. NTSR 저항성을 획득한 잡초는 다른 작용 기구를 가진 제초제에 대해서도 교차저항성을 발휘할 수 있으므로 TSR 저항성을 획득한 잡초보다 방제가 더 어렵다. 저항성을 획득한 잡초는 생장 적응을 위한 비용이 필요로 할 수도 있는데, 이러한 적응 비용을 다발성 발현 효과라 한다. 적응 비용과 관련하여 저항성 식물은 감수성 식물에 비해 종자 및 biomass의 생산, 경합 능력 및 발아 등이 불리하거나 드물게는 유리할 수도 있다. 2017년 우리나라에서 ALS 저해 제초제에 대한 저항성 논 잡초의 발생 면적은 2012년에 비해 벼 재배면적 기준으로 약 2.8배 이상 증가하였다. 10,000ha 이상 발생한 저항성 잡초 종도 2004년 3개, 2008년 4개, 2012년 7개, 2017년 8개로 증가하였다. 2012년까지는 100,000 ha 이상 발생한 저항성 잡초는 없었으나, 2017년에는 저항성 M. vaginalis, Echinochloa spp. 및 S. juncoides가 각각 292,936 ha, 180,753 ha 및 138,756 ha나 발생하였다. 2013-2015년에 걸쳐 전국에서 채집된 E. oryzicola에서도 ACCase 및 ALS 저해제에 대한 저항성 및 교차저항성이 발생하였다. ALS 저해제에 대한 저항성은 M. korsakowi의 경우 als 유전자의 주요 변이 부위인 197Pro(CCT)이 아닌 다른 부위의 변이에 의해서, M. vaginalis 및 S. trifolia에서는 197Pro(CCT)의 변이에 의해서 유기되었다. 또한, 일부 S. juncoides의 저항성 biotype도 197Pro의 변이에 의해 낮은 정도의 저항성을 나타냈다. 이와 같은 결과로부터 M. korsakowi, M. vaginalis, S. trifolia 및 일부 S. juncoides의 저항성은 TSR 메커니즘에 의해 유기된 것으로 사료되었다. 한편, 또 다른 저항성 S. juncoides GS28은 als 유전자의 돌연변이가 일어나지 않았음에도 불구하고 강한 저항성을 나타내었으나, P450의 저해제인 fenitrothion 처리에 의해 저항성이 현저하게 감소하였음으로, NTSR에 의해 저항성이 유기된 것으로 사료되었다. 저항성이 TSR에 의해 유기된 것으로 보이는 S. trifolia는 종자 발아율에서 감수성 biotype보다 유리한 생장 적합도를 나타내었으며, 종자에서 유래된 유식물의 생장도 촉진되었다. 그러나, 저항성 S. trifolia의 괴경에서 유래된 유식물은 생장이 억제되었음으로, 유식물의 생장에 있어서는 저항성 및 감수성 biotype간에 상반된 적합도를 나타내는 것으로 사료되었다. 현재까지 우리나라에서는 다수의 ALS 및 ACCase 저해 제초제 저항성 잡초의 발생이 보고되었다. 그러나, M. korsakowi, M. vaginalis, S. trifolia 및 S. juncoides에서만 target 단백질 유전자의 변이 여부가 밝혀졌다. 따라서 제초제 저항성의 구명을 위해서는 분자생물학적 연구를 통하여 더 많은 저항성 초종들에서 target 단백질의 돌연변이 여부를 밝히는 것이 필수적이다. 또한, 이러한 연구를 통하여 제초제 저항성 잡초의 발생을 방지하고 기 발생된 저항성 잡초의 방제에도 이용 가능한 대책을 마련하는 것이 필요하다.