INTRODUCTION
Soil is a key element for grass growth (Vescovo et al., 2004). Soil nutrition determines the health of grasses and inadequate nutrition may result in reduced foliar and root productivity (Fu and Huang, 2004; Lee, 2008). Turf cover is significant in that it modifies intrinsic soil properties (National Turfgrass Evaluation Program, 2009). Turf improves the micro climatic environment and supplies organic matter which amends the activity of microorganisms (Woods et al., 2006; Lynch and Brown, 2008). Turf also enhances nutrient availability, all of which are necessary for sustainable grass performance.
Many studies have been focused on shrubs with grasses receiving much lesser attention and
even less studies are focused on both above- and below-ground growth characteristics. Roots play a critical role in absorbing soil nutrition for foliar development. The presence of roots also provides soil with enhanced infiltration rates (McCarty et al., 2005), and water uptake (Spring et al., 2007).
Several authors (Carrow, 1980; Sherratt et al., 2005; Lee, 2008; Su et al., 2008) proposed that roots occupy available macro pore spaces and develop deep into the soil matrix seeking out air, water and nutrients. Su et al. (2008) and Vanini and Rogers (2008) reported that differences in root morphology, root diameter, root length, density, and their distribution within the soil will contribute to soil nutrition absorption. Fine lateral roots are the major sites of water and nutrient uptake. Lateral roots are fibrous and can establish very efficiently (White et al., 1993). They account for 90-98% of the total root length in a root system (Allen et al., 1998; Brosnan et al., 2005). Therefore, the density of lateral roots make up a significant proportion of the entire root system (Hays et al., 1991; Huang and Fry, 1998; Fu and Huang, 2004).
Although root architecture is somewhat genetically constrained, it still can be highly plastic in response to environmental cues. This is suggestive that genetically identical grasses may still differ in root density, growth rate, and distribution (Marcum et al., 1995; Qian and Fry, 1997; Huang and Gao, 2000), as a result of adaptive responses (Huang and Fry, 1998; Barton et al., 2009). For example, the heterogeneous distribution of nutrients can increase lateral root length as well as concentrate initiation of lateral roots in soil regions where resources are in rich supply (White et al., 1993; Gallardo et al., 1996). The localized application of nutrients at prescribed depths and associated lateral root development offers an opportunity to manipulate the distribution of these roots within the soil profile.
Deprivation of nitrogen (N) as well as phosphorus (P) often limits growth (Daniels and Sugden, 1996; Christians, 2004). Nitrate (NO3-) is the major source of N for most grasses and is readily available for uptake (Goss et al., 2002). Lynch and Brown (2008) and Ticconi and Abel (2004) proposed that the availability of P also assisted with increased lateral root extension while the effects of Potassium (K) were less pronounced (Cherney et al., 2004; Woods et al., 2006). Noteworthy also was that Tinker and Nye (2000) highlighted the importance of the presence of both nutrients, N and P in stimulating lateral root development.
In earlier work done by Levitt (1980) it was found that dense mats of lateral roots are common at the soil surface and the abundance of roots decline with increasing soil depth (Lawson, 1999; Liao et al., 2004). The abundance of lateral roots at deeper soil depths is beneficial, as it increases water and nutrient uptake (Lambers et al., 2006). Roots have been found to respond to heterogeneous nutrient supply with compensatory root growth (Lambers et al., 2002) in areas abundant with N or P (Lu et al., 1999; Ronnie et al., 2009). Furthermore, the proportion of lateral roots was also found to have increased in nutrient-enriched zones especially when calcium was present (Sartain, 1993; Spiers, 1993; Johnson et al., 2003).
In this paper, we compared rooting and foliar characteristics and their interaction with the soil between three turfgrass species. We identified the effects of soil nutrition on root properties with an emphasis on root proliferation such as lateral root growth. We also examined nutrient applications involving phosphate, nitrate, calcium, and potassium to stimulate root development. The objective of this study was to improve our understanding of the specific requirements of individual nutrients in providing for foliage and roots in three turfgrass species. Such understanding has significance in sports and recreational management as it provides knowledge to better grapple with fertilizer application. This information is useful as the requirements for fertilization in a recreational context are related to aesthetics. These have been achieved through the aboveground portions of the plant, but the absorption of the nutrients to enable the growth occurs predominantly below ground (through the roots).
MATERIALS AND METHODS
Experimental growth conditions
Zoysia matrella, Cynodon dactylon (Bermuda) and Stenotaphrum secundatum (St. Augustine) were grown in vertical columns comprised of clay loam soils made up of 40% clay, 25% loam, 20% sand and 15% organic matter (by volume). The pH was 7.5 and the column was divided into three compartments, one above another, separated by horizontal membranes. The aim was to inject nutrient solutions lacking a single nutrient into the top and bottom compartments of the column. The control (middle compartment) was given the complete nutrient solution whilst the top and bottom compartments received a solution deficient in a single nutrient. In doing so, we will be able to understand the response of roots to specific nutrient deficiencies.
Forty-five columns were built using Perspex measuring 80 cm in height and with a 30 cm internal diameter. A geotextile membrane was inserted 25 and another at 50 cm from the top of the column (Fig. 1). Nutrient solutions were fed into the system by an inlet built at fixed depths of 0, 25 and 50 cm and supplied to the split-root column at a rate of 2 ml/min. Excess or outflow was permitted through a separate outlet valve positioned at each compartment. Care was taken to ensure that each compartment was watertight. A complete randomised experimental design was used with 15 replicates per grass species and three replicates per nutrient treatment. Noteworthy also was that although the usual turfgrass rooting depth ranges from 5 to 30cm, we have allocated additional space in the split-root column to avoid any potential constraint conditions that might affect the outcomes of this study.
Turfgrass growing in trays obtained from the nursery were removed and placed into the top compartment of each column and kept under controlled (growth chamber) conditions. The chambers were set similar to equatorial tropical climates with a day and night time temperature of 29 and 23°C, respectively. The photoperiod was 12 hours. This growth process was maintained for 24 weeks. Thereafter, the grasses were excised and the columns decoupled so that the roots could be harvested, washed and separated into the various zones that corresponded to the three compartments of the split-root column.
St. Augustine grass was mowed weekly at 4.8 cm, whereas Bermuda and Zoysia grasses were mowed at 2.5 cm. All clippings were removed and harvested fortnightly and dried at 70°C for 24 hours prior to weighing. Turf performance which was regarded as turf quality was evaluated fortnightly on a 1 to 9 scale, where 6 was the minimum acceptable turf quality and 9 was the best. Turf coverage was rated as a percentage of area covered by living turf. Biomass measurements of foliage and roots were observed by placing the plant material in an oven set at 70°C for 48 hours. For the measurement of available N and P, the dried root samples were digested in a mixture of concentrated H2SO4 and H2O2. Digested samples were analysed for N by the Kjeldahl method and for P, using the molybdo-vanadophosphate method (Johnson and Ulrich, 1959; Shi, 1986; Da Silva et al., 2016).
Treatment and nutrient feeding
The experiment had four treatments (excluding controls) each with three replicates. Control plants had all the nutrients (NPK and calcium) supplied throughout the entire root system. The rest of the treatments received the complete nutrient solution (like the control) but was limited to the middle compartment. The top and bottom compartments were supplied with a solution deficient in a single nutrient either, N, P, K or calcium. The concentration of supplied nutrients was similar to the work done by Li et al. (2013) on urban and rural roadside soil cores. The nutrient concentrations were in the ranges of 41.5, 28.3, 15.3 and 10.4 mg kg-1 for N, P, K and calcium, respectively. Liquid fertilizer was developed according to methods detailed in Broschat (1995) and Catanzaro et al. (1998) and applied on a weekly basis. Outflowing nutrients were analysed on a weekly basis to ascertain leachate and depletion within each compartment and there were no significant chemical remnants observed in the outflow.
Data analysis
Factorial ANOVA was conducted across turfgrass species using SAS statistical software (SAS Inst., Version 9, NC, USA) for foliage, rooting and nutrient parameters. Significant differences between means (within the same species) were determined by LSD at the P≤0.05 probability level.
RESULTS
Dry matter distribution between foliage, roots and nutrient concentration (in roots)
The major effects of nutrient supply on foliage and root development are summarised in Table 1. Deficiency in phosphate, nitrate and calcium markedly reduced foliage biomass and clipping yield with a pronounced effect observed with nitrate deficiency. This finding was further reinforced through turfgrass coverage and turf quality ratings. Potassium deficiency was found to have significantly reduced foliage biomass but did not significantly affect root biomass. This outcome was especially pronounced in Zoysia matrella and the St. Augustine grass across all depths in the split-root column. The deficient supply of a given nutrient in the root was validated through the values of N and P uptake (Table 1). In addition, some interactions occurred between different nutrients; for example, a deficient supply of phosphate to the roots was also observed to have reduced the absorption potential of calcium (data not shown). Nutrient deficiency in calcium and phosphate resulted in broadly similar effects on root and foliage weight whereby, biomass data indicated that % difference in calcium and phosphate was between 0.9 to 7.5 and 2.9 to 7.4% for foliage and roots, respectively.
Specific nutrient deficiency on rooting characteristics
Root growth was most affected by the absence of nitrate where root biomass was reduced between 60 to 70% across the three species. However, roots were less affected by the absence of potassium (Table 1). The effects of phosphate and calcium deficiency on root growth were similar. These findings are consistent with data in similar studies conducted by Cherney et al. (2004) and Barton et al. (2006).
Comparative effects of phosphate, nitrate, potassium and calcium on root development
Generally, specific nutrient deficiency tends to elicit different responses in root proliferation but the broad effects are similar. The localised retardation of root growth was observed when all nutrients were omitted suggesting their importance in root development (data not shown). Noteworthy however, that potassium was a notable exception. Potassium was found to have the least impact on root development with biomass data indicating a small difference of between 2.8 to 5.9% across all three species when compared against the control. A localised deprivation of calcium elicited a similar response to that of phosphate, but it was nitrate that showed a pronounced slowdown in root development.
With the solution of NPK and Ca supplied, the control roots were lush all throughout the various compartments (Fig. 2). Nutrient deprivation clearly negatively affected root proliferation with nitrate particularly impactful (Fig. 2).
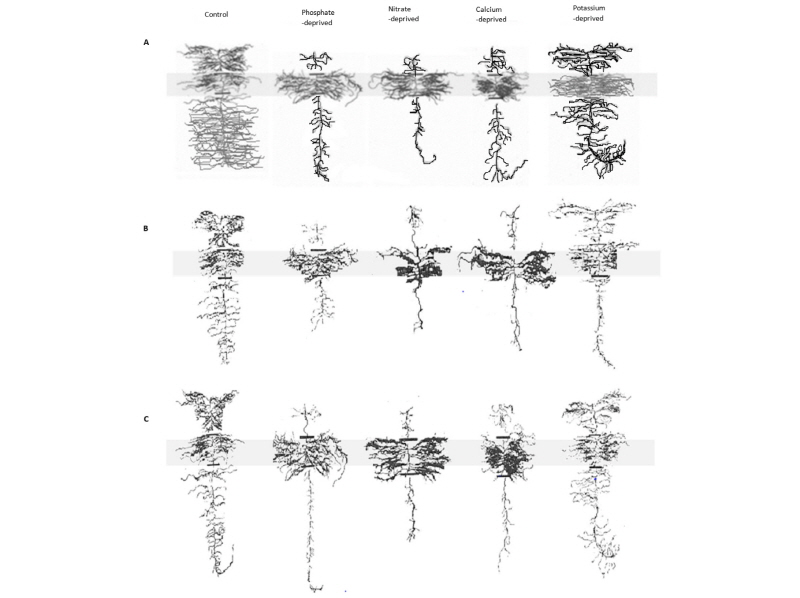
Fig. 2. Effect of localized supply of phosphate, nitrate, calcium, and potassium on root development in (A) Zoysia matrella, (B) Cynodon dactylon and (C) Stenotaphrum secundatum. Control received the complete nutrient solution to all parts of the root system. The others received the complete nutrient solution only in the middle shaded section, the top and bottom sections were supplied with a solution deficient in the specified nutrient.
DISCUSSION
This experiment was conducted in 2017 to characterize the effects of localized N, P, K supply in combination with calcium on root growth and nutrient uptake in common warm season turfgrass species. Turf is widely used for horticulture, recreational and sports purposes. Therefore, the results from this paper are significant as it provides information on suitable depth for fertilizer placement. It also validates targeted fertilization programs for horticulture, recreational and sports maintenance.
The results from this experiment demonstrated that considerable modification to overall dry matter distribution can occur in turfgrasses as a result of variation in the concentration of nitrate, phosphate, potassium and calcium within the rooting zone. Nitrate particularly had a pronounced effect on dry matter distribution. Similar physiological responses occurred when phosphorus and calcium were absent. Modifications to the root zone were most pronounced when nitrate was omitted and least pronounced when potassium was removed.
The localised effects on root distribution following deficiency of specific nutrients indicated that the presence of these nutrients in contact with the root system can directly affect the development of roots and foliage. Variation in the effects of root and foliage growth may occur between species (Vietor et al., 2004a) and genotypes (Flavel and Murphy, 2006). This may be attributed to differences in nutrient uptake and utilization efficiency (Vietor et al., 2002). Therefore for the purpose of comparison, future research may need to study this variability in cool season grasses. Nevertheless, root development is a low-cost strategy adopted by plants to absorb available ions, nutrients and water in heterogeneous environments. It requires only an additional 0.2% of daily carbon gained (Hodge, 2006). However, only roots with small diameters (i.e. fine roots) (Drew, 1975; Kirkham, 1983; Smucker, 1993; McCully, 1999) can take advantage of this as they face less resistance in the uptake of ions and water (Smucker, 1993).
Here in this study, potassium deprived solution was found to have the least impact on root growth both at shallower and greater depths. This finding was consistent with pioneering work conducted by Bengston and Davis (1939), as well as follow up work carried out by McClean and Watson (1985) and Cherney et al. (2004).
It is evident that nitrogen is essential for optimal growth in any part of the root system. Considerable amounts of this nutrient are translocated in the xylem to the shoot which explains the negative impact nitrogen deprivation had on foliage biomass. The contrasting outcomes between potassium and nitrogen in promoting the growth of roots may be attributed to the translocation abilities of nitrogen within the root system. Nitrogen had been proposed to move swiftly within the root system thereby, promoting optimum root development though there is still no strong evidence to suggest that nitrogen is more mobile than potassium within root tissues (Sims, 1990; McCoy, 1998). An alternative explanation is that very low concentrations of nitrogen in the roots are sufficient for growth (Reid, 1933; Linde and Hepner, 2005). This raises the question as to whether there is a threshold concentration for key nutrients. Exceeding which, increasing concentrations are no longer beneficial for root and foliage growth. Such questions pave the way for future research seeking out concentration threshold limits in common horticulture soils. The development (e.g. length, density) and distribution of roots in the soil will determine overall plant growth and sustainability. It had been argued that a higher rooting density and spread would be particularly beneficial in enhancing nutrient assimilation and that fertilizer placement may be one way of achieving this ideal outcome.
Several aspects of the findings made in this controlled experiment can be applied to field conditions. Firstly, all other nutrients examined in this study except potassium had a significant role in the development of roots. This finding was consistent with work carried out by Puhalla et al. (1999), Nikolai (2002) and Vietor et al. (2004b). Secondly, changes in root development may be nutrient specific - this reinforces the need to ensure that all critical nutrients are worked into the soil during fertilization for the best results. Thirdly, surface application of nutrients is likely to limit the benefits of root proliferation to shallower soil depths. To achieve a deep and well-spread root system, all nutrients should be mixed into the soil to depths as deep as possible (Carrow et al., 2004; Woods et al., 2006). In the case of an established landscape, the option of liquid injection of fertilisers may be an option to achieve deeper penetration of soluble nutrients.
CONCLUSION
Though this research was conducted under controlled conditions, the outcomes have relevance across other turfgrass species and field environments. More importantly, such information is valuable to municipalities, growers and maintenance personnel involved with horticulture, sports and recreational management. The literature holds very limited information on nutritional effects on root development relating to lawns for horticulture, sports and recreational purposes. This work had shown the key roles of various nutrients in root development with nitrogen possessing the most pronounced effect on root proliferation. Future research is best targeted towards the identification of localized application of key nutrients at specific soil depths and involving different soil types. Additionally, the establishment of concentration threshold limits for specific nutrients will also need to be identified. Related work may consider the responsiveness of different grass species though no significant differences were observed between the different grasses examined here. All three species responded similarly to the deprivation of the various nutrients. Targeted fertilization programs have environmental benefits because they facilitate the use of less chemicals and potentially have cost saving benefits and can prevent leachate and contamination.