Introduction
Sagittaria trifolia L. also known as three-leaf arrowhead, is a perennial monocot-weed belonging to Alismaceae. It is widely distributed in ponds, marshlands, and paddy fields in Asia (Chen et al., 2007; Daimon et al., 2014; Iwakami et al., 2014). It propagates mainly by underground tubers and produces numerous seeds by self-pollination and outcrossing (Chung et al.,1998; Ito, 1997; Kwon et al., 2006; Wang and Chen, 2000). This species is one of the most noxious weeds in paddy fields of Korea. Loss of yield of rice has been observed with increasing density of S. trifolia (Itoh and Miyahara, 1988; Song et al., 2006). Acetolactate synthase (ALS) inhibiting herbicides such as bensulfuron-methyl, penoxsulam, and pyribenzoxim are widely applied to control S. trifolia in paddy fields (Heap, 2018). However, excessive and continuous application of ALS inhibitors has led to the evolution of resistance in S. trifolia. To date, ALS-herbicide-resistant S. trifolia has been reported in Japan, China, and Korea (Heap, 2018). Sulfonylureas-resistant S. trifolia was firstly found in 1997 in Akita of Japan (Heap, 2018). This R biotype exhibited high levels of resistance to bensulfuron-methyl and pyrazosulfuron-ethyl due to an amino acid substitution at Pro197-Ser of ALS enzyme (Iwakami et al., 2014). In China, the R biotype of S. trifolia was firstly identified in a paddy field of Liaoning province in 2014 (Heap, 2018). This R biotype had an als gene mutation at Pro197-Leu which conferred high resistance to bensulfuron-methyl (Wei et al., 2015). In Korea, the first R biotype was identified in a paddy field of Gyeongsang province in 2011 (Heap, 2018; Park et al., 2014). However, the physiology and molecular basis of resistant to ALS inhibitors for this biotype remain unknown.
The fitness of weeds is a quantitative feature of the adaptation of weeds to the environment. Evolution and dynamic acclimatization play an important role in influencing the fitness of weeds in the natural environment (Athanasiou et al., 2010). Differential fitness of R and S weeds is an important factor affecting the evolution of herbicide-resistance (Vila-Aiub et al., 2009). Germination, growth, reproductive capacity, and competitiveness of plants are usually used to assess differences of fitness between herbicide-resistant and susceptible weeds (Holt, 1988; Warwick, 1991). It is worth noting that gene mutation of weeds endowing resistance to ALS inhibitors probably has a negative effect on the fitness (Donnelly and Hume, 1984; Hobbs, 1987; Jacobs et al., 1988; Menchari et al., 2008; Wu et al., 2018). Especially, although target-gene mutation of weeds can confer resistance to herbicides, such mutation might have an adverse effect on the fitness of herbicide-resistant biotypes such as reduced photosynthesis, biomass accumulation, and reproductive output. It has been shown that seed production of herbicide-resistant Arabidopsis thaliana with Pro197-Ser mutation is significantly decreased compared to that of its susceptible biotype (Purrington and Bergelson, 1997). On the other hand, herbicide-resistant Setaria mutant with gene mutation has higher fitness such as more vigorous and earlier growth than its susceptible biotypes (Wang et al., 2010). Therefore, it is necessary to understand the difference in fitness between herbicide-resistant and susceptible S. trifolia.
Thus, the objectives of the present study were: 1) to determine the resistance-level of S. trifolia biotypes to ALS inhibitors, 2) to investigate the molecular basis of resistance mechanism in S. trifolia biotypes, 3) to understand the difference in fitness between R and S biotypes. The ultimate purpose of this study was to estimate population dynamics of R biotypes in the paddy field.
Materials and methods
Samples source
Tubers of suspected herbicide-resistant (R) S. trifolia were collected from an infested paddy field of Gyeongsangnam-do, Korea. One susceptible (S) S. trifolia was collected from Chungnam province. These tuber samples were stored in a refrigerator at 4℃ for longer than one month. For seed production, R biotypes grown from tubers were separated to avoid outcrossing because their outcrossing nature might lead to high number of polymorphisms (Larran et al., 2017). Collected seeds in greenhouse were air-dried and kept in the refrigerator at 4℃.
Dose-response test
Tubers of the R and S biotypes were placed in an incubator at 28℃ for four days to accelerate germination. Five plants were respectively grown in pot (L: 20 cm, W: 14 cm, H: 11.5 cm) filled with 5 cm of paddy soil. Three ALS-inhibiting herbicides cyclosulfamuron, penoxsulam, and imazaquin were applied to plants at 5-leaf stage. Cyclosulfamuron was applied at 0.19-480 g a.i. ha-1 to S biotypes and at 1.9-7,680 g a.i. ha-1 to R biotypes. Penoxsulam was applied at 0.015-150 g a.i. ha-1 to S biotypes and at 0.015-4,800 g a.i. ha-1 to R biotypes. Imazaquin was applied at 3.1-6,400 g a.i. ha-1 to both S and R biotypes. Fresh weights of shoots were measured at 20 days after treatment.
The experiment was conducted in a completely randomized design with three replicates. Data were analyzed using a non-linear regression equation as follows:
(1)
In this equation, y was fresh weight, x was the dose of ALS-inhibiting herbicide, a was the minimum value, d was the maximum value, c was GR50 (the herbicide dose required for 50% biomass reduction compared with the untreated), and b was proportional to the slope around the dose of GR50.
als gene sequencing
The newest leaves (100 mg) were cut from R and S plants. DNAs of R and S plants were extracted from these leaves using a DNeasy® Plant Mini Kit (QIAGEN, DE, USA) according to the manufacturer’s protocol (Anonymous, 2017). The concentration of DNA was measured using a NanoDrop 2000 spectrophotometer (Thermo Scientific, Waltham, USA) (Anonymous, 2009). Subsequently, polymerase chain reaction (PCR) was performed to amplify DNA fragments with a pair of primers: 5'-ACTCCGCCATCACCT-3' (sense primer, F1) and 5'-GGGAAACTAAGAGCGCAT-3' (antisense primer, R1) (Iwakami et al., 2014). PCR was performed for 35 cycles of 95℃ for 30 s, 60℃ for 30 s, and 72℃ for 2 min following an initial denaturation at 95℃ for 15 min. The PCR was finished with a final extension step for 10 min at 72℃. DNA fragments encoding a portion of als gene were also amplified by PCR using another pair of primers: 5'-GTTCCCATGCCACTGAAGAC-3' (sense primer, F2) and 5'-ATCGCATCACCGAACTTCTT-3' (antisense primer, R2). PCR was performed with primers F2 & R2 for 38 cycles of 95℃ for 20 s, 64℃ for 30 s, and 72℃ for 80 s following an initial denaturation at 95℃ for 2 min. The final extension step was performed at 72℃ for 5 min. To detect the mutation site of als gene between R and S plants, PCR products of als genes were sequenced. Alignment of DNA sequences was performed using a DNAMAN program (LynnonBiosoft, San Ramon, USA).
Fitness test
Seed germination rates of R and S biotypes were determined under light and dark conditions in an incubator with constant temperature of 28℃. Fifty seeds of each biotype were placed on Whatman No. 2 filter papers in a Petri dish (φ: 9 cm, H: 1.5 cm). In each Petri dish, 3 mL of distilled water was added. The number of germinated seeds was counted at 24 hours after sowing and the rate of seed germination was calculated for each biotype. The experiment was conducted with five replicates.
Plant growths after cultivation of seeds were compared between R and S biotypes. Seeds of R and S biotypes were vernalized in an incubator at constant temperature of 28℃ as described above. Five days after sowing (DAS), five seedlings for R and S biotypes were respectively transplanted into pot (L: 20 cm, W: 14 cm, H: 11.5 cm) containing 5 cm of paddy soil. At 20 days after transplanting (DAT), plants were washed and then photographed with a camera (Canon100D, Tokyo, Japan) to compare growth between R and S biotypes. Plant height and fresh weight were measured at 20 DAT. The experiment was repeated four times.
Growth of plant after cultivation of tubers was also compared between R and S biotypes. Tubers of R and S biotypes were incubated at constant temperature of 28℃ for fourteen days. Five tubers for R and S biotypes were respectively transplanted into pots as described above. Bud and growth of plants were photographed at 20 DAT. Plant height and fresh weight were also measured at 20 DAT. The experiment was repeated four times.
Results
Dose-response test
Whole plant dose-response test was conducted to determine resistance levels of S. trifolia to three ALS inhibiting herbicides (cyclosulfamuron, penoxsulam, and imazaquin). The R biotypes originated from tubers were resistant to three ALS inhibiting herbicides (Fig. 1). GR50 values of the R biotypes to cyclosulfamuron, penoxsulam, and imazaquin were 794, 300, and 196 g a.i. ha-1, respectively. Meanwhile, GR50 values of the S biotypes to cyclosulfamuron, penoxsulam, and imazaquin were 3.2, 23.5 and 23.6 g a.i. ha-1, respectively. Thus, resistance levels for the R biotypes to cyclosulfamuron (sulfonylurea), penoxsulam (triazolopyrimidine), and imazaquin (imidazolinone) were 250, 13, and 8, respectively (Table 1).
als gene sequencing
Partial als genes of R and S biotypes were sequenced with a length of approximately 2,060 bp. These sequences included a 1,830 bp region that encoded a protein of 610 amino acid residues. Nucleotide sequences of als gene of R and S biotypes exhibited 99.95% similarity. Based on sequence comparison, a single-point mutation from C to T at nucleotide 410 leading to amino acid substitution from proline (CCC) to leucine (CTC) at position 197 in the R biotype was found (Fig. 2). No gene mutation was observed in the R biotype at position of Ala122, Ala205, Asp376, Arg377, Trp574, Ser653, or Gly654 of the als gene, although these mutations have been reported in herbicide-resistant biotypes of other weed species (Corbett and Tardif 2006).
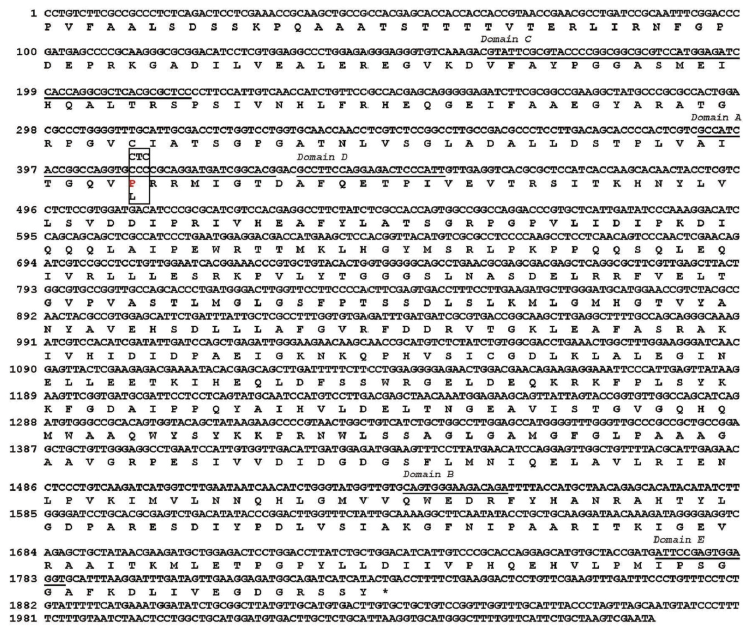
Fig. 2. Nucleotide and deduced amino acid sequences of als gene from herbicide susceptible (S) and resistant (R) S. trifolia biotypes. The underlined squence indicates highly conserved regions (domains A, B, C, D, E) in plant als gene. The box indicates a Pro to Leu mutation at amino acid 197 in domain A in the R biotype. The number of amino acids is based on the precursor acetolactate synthase (ALS)-gene from Arabidopsis thaliana. GenBank accession number is AB301496.1 for wild type als gene of S. trifolia.
Fitness test
Under light condition, the maximum germination rates of R and S biotypes were 18% and 4%, respectively. The maximum germination of the R biotypes was observed at 10 DAS while that of the S biotypes was found at 5 DAS (Fig. 3). Under dark condition, the maximum germination rates of R and S biotypes were 11.6% and 4.0%, respectively. The maximum germination rate of the R biotypes in the dark condition was lower than that in the light condition (Fig. 3).
Although evolved resistance mechanisms in weed species can protect them under various stresses, they may have fitness cost. The fitness cost was unique in growth and development process of S. trifolia in this experiment. S. trifolia biotypes having target site resistance demonstrated unpredicted fitness costs, showing diverse results for plants originated from seeds and tubers (Fig. 4). S biotypes originated from seeds showed slower growth with average plant height of 1.95 cm whereas R biotypes showed vigorous growth with average plant height of 2.99 cm. On the contrary, S biotypes originated from tubers exhibited greater vegetative growth with average plant height of 14.75 cm whereas R biotypes showed smaller vegetative growth with plant height of 6.85 cm (Fig. 5).
Similar results were observed for fresh weight parameter of S. trifolia of both S and R biotypes. The fresh weight of the S biotypes originated from seeds was 0.28 g compared to 0.68 g for the R biotypes. Fresh weights of S and R biotypes originated from tubers were 2.24 g and 1.04 g, respectively. Interestingly, both S and R biotypes of S. trifolia originated from tubers exhibited greater performance compared to those originated from seeds. It is likely that fitness cost in S. trifolia is conferred by target site resistance.
Discussion
In this study, the R biotypes originated from tubers were highly resistant to cyclosulfamuron (sulfonylurea) and moderately resistant to penoxsulam (triazolopyrimidine) and imazaquin (imidazolinone) (Fig. 1; Table 1). Iwakami et al. (2014) have reported that the R biotype of S. trifolia collected from the paddy field in Akita of Japan has evolved high levels of resistance to bensulfuron-methyl and pyrazosulfuron-ethyl (sulfonylureas) (R/S: 460 and 100, respectively). Two R biotypes of S. trifolia from Liaoning province of China were also highly resistant to bensulfuron-methyl (sulfonylureas), with R/S ratios of 76.99 and 49.94, respectively (Wei et al., 2015). In addition, cyclosulfamuron-resistant weeds exhibited cross-resistance to other ALS inhibiting herbicides. For example, cyclosulfamuron-resistant Cyperus difformis and Rotala indica were found to have cross-resistance to bensulfuron-methyl, bispyribac-sodium, and imazapyr (Kuk et al., 2002; 2004). The occurrence of cross-resistance was probably related to the mechanism of resistance, especially target site-based resistance mechanism.
Target site-based resistance mechanism was investigated in R biotypes originated from tubers in this study. An amino acid substitution from Pro197 to Leu197 was identified in the R biotype of the present study (Fig. 2). Proline to Leucine mutation at position of als gene 197 conferring high resistance to ALS inhibitors in R biotype of S. trifolia in China has also been reported (Wei et al., 2015). Proline substitutions at position of als gene 197, such as Pro197-Ser, -His, and -Thr, are common mutations that can confer resistance to ALS inhibitors in S. trifolia of Japan and China (Fu et al., 2017; Iwakami et al., 2014). In addition, non-target site-based resistance mechanism in the S. trifolia of Japan and China has been previously reported. A molecular basis of ALS inhibitors-resistance in S. trifolia has been previously investigated through genomic DNA Southern blot analysis, suggesting that R biotype has a non-target-site-based resistance mechanism (Iwakami et al., 2014). Regarding metabolism-based resistance to ALS inhibitors in S. trifolia, it has been previously reported that penoxsulam application with malathion could effectively inhibit dry weight accumulation of R biotype than treatment without malathion (Zhao et al., 2017). In the present study, we just found the target-based resistant R biotype of S. trifolia.
The fitness of herbicide-resistant weeds is generally associated with the generating mechanism of resistance (Elam et al., 2007). Especially, target-gene mutation of weeds conferring resistance to herbicide probably has positive, negative, or no effects on plant fitness of phenotypes. It has been reported that sethoxydim-resistant Setaria mutant with Ile1781-Leu mutation of accase gene exhibits earlier flowering, vigorous growth, and more tillers than its S biotype (Wang et al., 2010). In contrast with results from Vila-Aiub et al. (2005) research, seed germination and seedling emergence of ACCase inhibitors-resistant Lolium rigidum containing Ile1781-Leu mutation of accase gene were significantly less than those of the S biotype. Vercellino et al. (2018) have reported that metsulfuron-methyl-resistant Raphanus sativus with Trp574-Leu mutation of als gene shows 22-38% and 21-47% lower seed numbers and yield per plant than the S biotype, respectively. In this study, we first investigated the relative fitness of R and S biotypes of S. trifolia originated from seeds. Germination and growth of the R biotype with the Pro197-Leu mutation of als gene were greater than those of the S biotype (Fig. 3 and Fig. 5), indicating that the target-gene mutation (Pro197-Leu) of als gene might have positive effects on fitness of S. trifolia.
Differential fitness of R and S biotypes has been identified as a key factor influencing the evolution of herbicide-resistance (Warwick and Lynn, 1994). In absence of selection-pressure of herbicide, if R biotypes are more fit than S biotypes, R biotypes will be dominant in the population. R biotypes of S. trifolia originated from seeds might become dominant in the population of uninfected paddy fields because the growth of R biotypes was more vigorous than that of the S biotypes (Fig. 5). Similar reported the growth of glyphosate-resistant Eleusine indica and triazine-resistant Amaranthus blitoides was more vigorous than that of the S biotypes (Ismail et al., 2002; Sibony et al., 2003). Conversely, when S biotypes are more fit than R biotypes in absence of selection-pressure of herbicide, S biotypes will become dominant in the population. In this study, the growth of the S biotypes originated from tubers was more vigorous than that of the R biotypes (Fig. 6), indicating that the S biotype will probably become dominant in the population in the absence of herbicide. Similar results have been observed for susceptible Senecio vulgaris whose competitive capacity is greater than atrazine-resistant biotype (Conard and Radosevich, 1979). Although the fitness of R biotype was weaker than that of S biotype, R biotypes might still become dominant in the population because S biotype will be killed by herbicide application.
In conclusion, the DNA of R biotypes had a mutation at the position of Pro197 to Leu in the als gene which conferred high resistance to ALS inhibiting herbicide. Once seeds of R biotypes are moved to uninfested paddy fields, the R biotypes containing als gene mutation will exhibit greater germination than existing S biotypes. Thus, the R biotypes will probably become dominant in the population. If ALS inhibitors are applied in paddy fields, the R biotypes will survive and even produce tubers which will continually propagate in uninfected paddy fields. To control the R biotypes, increasing the application rate of ALS inhibitor will probably enhance ALS-inhibitors’ selection-pressure.
Acknowledgement
This work was carried out with the support of "Cooperative Research Program for Agriculture Science & Technology Development (Project No. PJ01245703)" funded by Rural Development Administration, Republic of Korea.
Authors Information
Jia WeiQiang, http://orcid.org/0000-0002-1654-3638
Sug-Won Roh, Rural Development Administration Food Crop Industry Technology Service Division, Researcher
SungHwan Choi, Gyeongnam National University of Science and Technology, Researcher
Jong Chan Won, Gyeongsang National University, Researcher
Soo Yong Shim, Gyeongsang National University, Researcher
Jung-Sung Chung, Gyeongsang National University, Researcher
Le Thi Hien, Chungnam National University, Researcher
Aung Bo Bo, http://orcid.org/0000-0001-7579-3429
Kee Woong Park, http://orcid.org/0000-0003-0053-9543
Jeung Joo Lee, Gyeongsang National University, Professor