Introduction
Iron is an essential nutrient for living organisms because it has important functions in many cellular processes; however, excess soluble iron in plant cells leads to the formation of harmful hydroxyl radicals that can negatively affect proteins, membranes and metabolic pathways (Verbon et al., 2017). For decades, iron has been used in foliar applications for purposes such as turfgrass colour enhancement and to address iron deficiency (Wehner, 1992). Both ferrous sulfate and ferric sulfate can be used to increase chlorophyll production in plants, resulting in deeper green foliage (Yust et al., 1984; Havlin et al., 2005). Ferrous sulfate is the common source of iron for turfgrass management (Havlin et al., 2005). More recently iron has been investigated for another purpose, turfgrass disease prevention. Some research has shown the ability of ferrous sulfate to act as a control for turfgrass diseases such as dollar spot (Reams, 2013; McCall et al., 2017), and Microdochium patch (Mattox et al., 2017; Mattox et al., 2023), and against mosses (Burnell et al., 2004), although it has generally not worked against turfgrass snow molds (Koch and Hockemeyer, 2021), and also causes issues with turfgrass phytotoxicity and thinning (Mattox et al., 2020; Hsiang et al., 2022).
Iron Uptake and Transport
The main mode of iron acquisition by plants is direct uptake through the rhizosphere (Morrissey and Guerinot, 2009). Under normal growth conditions, where iron is not deficient, plant roots are able to reduce ferric (Fe+3) chelates present in the soil into soluble ferrous (Fe+2) ions. Ferrous ions can then be readily taken up through the plasma membrane due to their soluble nature (Briat and Lobreaux, 1997). However, the soil redox potential and the alkalinity of the soil affect iron availability and uptake in the soil (Morrissey and Guerinot, 2009). If the soil pH is high and under aerobic conditions, then most iron in the soil will become insoluble ferric oxides (Morrissey and Guerinot, 2009). However, if the soil is acidic, then iron is reduced into the soluble ferrous ions, which can then be transported into the epidermal cells of the roots (Morrissey and Guerinot, 2009).Once inside the epidermis of the root, iron undergoes chelation with citrate before transport through the xylem (Kim and Guerinot, 2007; Morrissey and Guerinot, 2009). Without this, iron would precipitate on apoplast walls (Morrissey and Guerinot, 2009). Although iron can be transported in the xylem, phloem transport is more efficient at transporting iron to growth areas of the plant (Kim and Guerinot, 2007). Since phloem sap has a pH greater than 7, ferric and ferrous ions form a chelate with nicotianamine to prevent it from precipitating and becoming insoluble (Kim and Guerinot, 2007; Morrissey and Guerinot, 2009). Once iron has reached the intended target cells, iron must then be transported across the plasma membrane using the ferrous ion transporter known as iron reductase (Lucena, 2008). Heavy rates of ferrous sulphate applications can lead to deposits in leaf cells (Fig. 1).Foliar Applications of Iron \
Iron salts have been used in foliar applications for over 150 years, to improve plant health and yield (Chen and Barak, 1982). Foliar applications of iron are made to address iron deficiencies or to achieve a greening effect (Hull, 1999). Eddings and Brown (1967) found that iron absorption was significantly higher during the day versus night, indicating the importance of open stomata. Among plants examined, the poaceous Sorghum had the highest rate of translocation (60%), which may be due to veinal and stomatal patterns in the leaf. Poaceae species have stomata aligned in rows, with veins running in between, and this allows short transport distance from stomata to conductive tissues, and more iron to reach the phloem for translocation throughout the plant (Eddings and Brown, 1967). However, even with this vein and stomatal pattern, turfgrass species are still relatively inefficient at translocating iron out of the treated leaf blade (Hull, 1999). Mixing a surfactant with the iron solution can significantly increase the amount of iron absorbed by the plant, since surfactants likely allow the iron solution to pass through the stomatal opening because of reduced surface tension (Eddings and Brown, 1967). Intensively managed turfgrass receives frequent mowing, which eliminates the area of the leaf that receives the majority of the foliar iron application. However, some of this solution will be washed into the top layer of the soil, where roots can absorb it and transport it throughout the plant (Hull, 1999). The efficiency of this process is dependent on many environmental factors such as soil pH, and hence frequent foliar applications are generally necessary to keep the desired effect from the iron applications (Hull, 1999).Iron sulfate and discoloration
Discoloration and even phytoxicity have been observed with applications of ferrous sulfate to turfgrasses. A foliar iron application to turfgrass causes a varying degree of darkening or blackening to the leaf blades following application. Darkening effects can be observed within 1 hour (Rudland, 2020) or 2 hours of iron application (Wheeler et al., 1985). Iron has been previously shown to have an essential role in chlorophyll production, acting as an enzyme cofactor (Lee et al., 1996). By adding more iron to a plant system, chlorophyll production is stimulated, which results in a dark greening effect, due to the increased chlorophyll and chloroplast numbers (Lee et al., 1996). Yuan et al. (2018) applied various iron treatments to chili pepper leaves to investigate this relationship. After ferrous sulfate was applied, chlorophyll content significantly increased by three-fold and the number of chloroplasts per cell increased 2 fold compared to untreated leaves, resulting in a greater density of chloroplasts, with a higher chlorophyll concentration in each chloroplast. Similarly, Lee et al. (1996) found a significant positive correlation between the amount of foliar iron applied and leaf blade darkening in Kentucky bluegrass (Poa pratensis). They found that, on average, chloroplast membrane volume increased up to 24% in Kentucky bluegrass exhibiting darkening, and furthermore that intense darkening can also be associated with phytotoxic effects (Lee et al., 1996).An experiment conducted by El-Jendoubi et al. (2014) on peach tree leaves showed that ferrous sulfate only caused a greening effect in the areas of direct contact, with some translocation to the leaf tip, but none towards the basal portion of the leaf. They only used low concentrations of ferrous sulfate, so higher concentrations may be able to translocate further away from the treated area. However, when treated leaves were examined under a microscope, large pools of iron were found in the treated areas, causing a blackening effect. This indicated that there are difficulties in moving the iron once it is inside the treated area as it may collect instead of moving throughout the entire leaf (El-Jendoubi et al., 2014). Therefore, the increase in chlorophyll and chloroplasts results in a greening effect, whereas a build-up of iron likely causes a blackening effect (El-Jendoubi et al., 2014).Yust et al. (1984) conducted a study on Kentucky bluegrass to determine the amount of foliarly applied iron causing phytotoxicity. They showed that phytotoxic symptoms (foliar dieback) began appearing at 17.7 kg ha-1 Fe (equivalent to 84.3 kg ha-1 of ferrous sulfate). However, iron application in the range of 4.5 to 17.7 kg ha-1 Fe only caused a darkening effect and the grass could recover. Rudland (2020) tested darkening in field trials, and observed that after one hour, darkening was especially noticeable for higher rates of ferrous sulphate ( > 125 kg ha-1), and for 25 kg ha-1, the darkening peaked at 24 hours after treatment, and dissipated over the course of a week. For the higher rate tested ( >125 kg ha-1), the darkening was still noticeable at 28 days compared to the untreated control. In general, there was an increase in darkening levels and duration as ferrous sulfate rates increased, as well as with shortened application intervals. In lab tests, rates from 25 kg ha-1 to 2500 kg ha-1 showed that the lower rates gave darkening while the higher rates showed phytotoxicity (Fig. 2). In field tests, the darkening was strongest after 1 day, and decreased after 7 days to just above background untreated levels for 25 kg ha-1 while the 125 kg ha-1 rate still was noticeable (Fig. 3) in mid-June. A similar field experiment in early August showed phytotoxicity at 125 kg ha-1 rate.Lastly, another point regarding the blackening effect observed is that different forms of iron can stain and accumulate on metal and plastic surfaces, and they darken turfgrass at different intensities. Rudland (2020) and Stone (2019) found that ferrous sulfate stains metal, plastic and grass three times more than ferric sulfate.
Iron sulfate and dollar spot
Hsiang (2016) conducted preliminary trials to investigate effects of lower risk fungicides against dollar spot. One of the selected compounds was ferrous sulfate heptahydrate, which was applied weekly to plots grown on putting greens comprised of a mix of P. annua and Agrostis stolonifera. Overall, ferrous sulfate significantly reduced disease compared to the inoculated check in those tests. Hsiang (2016) only tested 100 mM (15.2 kg ha-1 ) in the field. Stone (2019) tested ferric sulfate and found that that optimal rate for suppression dollar spot disease on Agrostis stolonifera was 261 g 100 m-2 (73 g Fe 100 m-2), and Rudland (2020) found that 52.5 g Fe 100 m-2 (in ferrous sulfate) significantly reduced dollar spot disease in field trials by 75% on average.
McCall et al. (2017) were the first group of researchers to compare the effect of ferrous and the elemental components, Fe and S against dollar spot. The purpose of this study was to determine if Fe, S or both elements could aid in the reduction of dollar spot disease. Biweekly applications of ferrous sulfate (48.8 kg ha-1), iron chelate (Fe-EDTA) (11.2 kg Fe ha-1) and sulfur (10.3 kg ha-1) were made from May to September in 2012 and 2015 against dollar spot. In both years, ferrous sulfate significantly reduced disease but elemental sulfur had no apparent effects on disease. These results agreed with Reams (2013), who also tested the elemental components of ferrous sulfate against dollar spot, including ferrous sulfate, iron chelate and sulfur. He found that ferrous sulfate significantly reduced dollar spot counts compared to sulfur and the inoculated control, but this did not significantly differ from the iron chelate treatment. Sulfur did not significantly differ from the inoculated control, and the iron chelate had significantly less dollar spot compared to the sulfur treatment and inoculated control. Both studies suggest that ferrous sulfate has the greatest disease suppression and that the iron component is responsible for the observed suppression of dollar spot disease (McCall et al., 2017; Reams, 2013).
Shelton (2018) conducted a field study on Agrostis stolonifera of increasing rates of ferrous sulfate (20% Fe) against Clarireedia jacksonii, and they estimated that 26 kg ha-1 and 100 kg ha-1 would result in 50 and 75% disease reduction. Rudland (2020) conducted field efficacy trials of ferrous sulfate across five creeping bentgrass cultivars, and found that the 25 kg ha-1 and the 125 kg ha-1 treatments had average disease suppression of 74% and 95%, respectively, within one week of repeated applications. Similarly, in a 2019 efficacy trial (across 10 cultivars), these rate had average disease suppression of 78% and 98%, respectively, within one week of repeated applications, and the higher rate had statistically similar levels of disease suppression compared to the fungicide control, Banner Maxx at at 26 ml 100 m-2. In the 2018 trial, efficacy remained stable until the end of the trial, whereas in the 2019 trial, the 1x efficacy dropped to 43% by two weeks after the last treatment, but the 5x efficacy remained stable until four weeks after last treatment, where it dropped to 79% suppression. Rudland (2020) hypothesized that the time of year (Fall 2018) allowed for a 4-week residual efficacy after the last 1x ferrous sulfate treatment because conditions were less favourable for disease development, whereas in Summer 2019, the residual efficacy of the 1x treatment was only up to two weeks long, perhaps because of favourable disease development conditions. Higher levels of iron in leaf tissue cells may be promoting more frequent and longer lasting oxidative bursts, which helps the plant fight off the pathogen for a longer duration (Verbon et al., 2017). Fig. 4 shows the effect of ferrous sulfate application on dollar spot disease at different intervals (3.5, 7 and 14 days application intervals) and at different rates (25, 50 and 75 kg ha-1). The inoculated control showed high levels of disease while the ferrous sulphate at 25 kg ha-1 gave the highest level of control without leaving scars from phytotoxicity.
Fig. 4
Plots of ‘Penncross’ creeping bentgrass treated repeatedly with FSH (ferrous sulfate heptahydrate) at 3.5, 7 or 14 day intervals with rates of 1x (25 kg ha-1), 2x (50 kg ha-1) and 3x (75 kg ha-1) starting in mid-June until pictures taken in July at 14 days after the last treatment, and 35 days since inoculation. All plots were inoculated with the Clarireedia jacksonii at 1 g m-2.
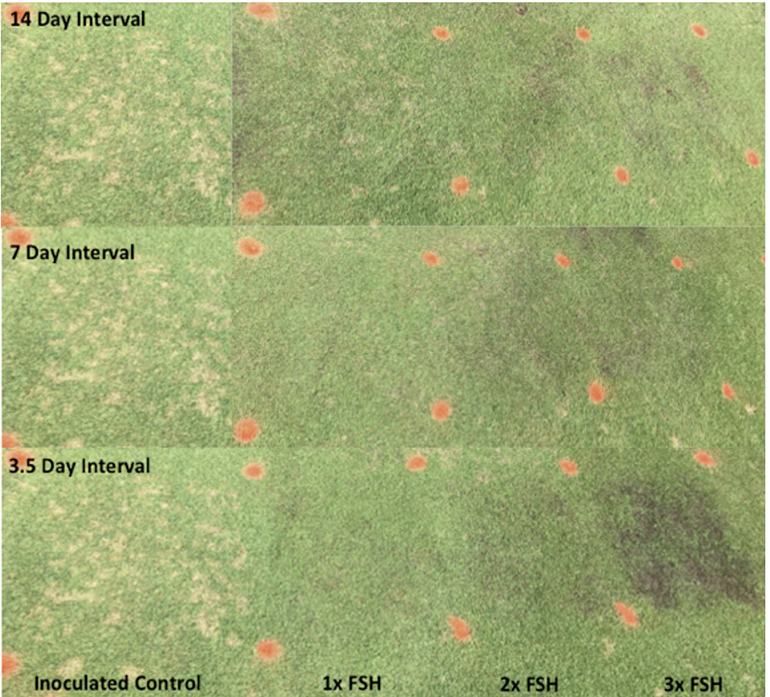
Rudland (2020) compared different cultivars of Agrostis stolonifera for their reaction to ferrous sulfate. Out of the five cultivars examined in 2018, Alpha responded the least to 1x ferrous sulfate treatment, whereas 007 and Tyee responded the most, by showing the greatest decrease in disease levels compared to the inoculated control (Rudland, 2020). In the 2019 trial, Alpha, MacKenzie, V8 and Focus responded the least, whereas L93 and 007 responded the most, indicating that cultivars of Agrostis stolonifera differ in their response to ferrous sulfate treatment against dollar spot. Rudland (2020) also found that there were no differences for individual cultivars in their response at greens height (5 mm) or fairway height (10 mm).
Physiological effects of iron sulfate
Overall, iron can be toxic in large quantities by stimulating the production of ROS (reactive oxygen species), which can then damage cell membranes through lipid peroxidation (Mahender et al., 2019; Rout and Sahoo, 2015). ROS are a normal part of the plant system, but when they accumulate freely in high quantities inside a cell, they can cause cell death (Dixon and Stockwell, 2014). Common above-ground symptoms of iron toxicity include growth reduction of the leaf blades, wilting of shoots, leaf blade yellowing or blackening and complete tissue necrosis (Mahender et al., 2019; Rout and Sahoo, 2015). Phytotoxic effects also occur below ground including blackening and stunting of the roots, as well as a reduction in root branching and increased flaccidity (Rout and Sahoo, 2015; Wheeler et al., 1985). Rudland (2020) tested root toxicity of different rates of ferrous sulfate (25, 125, 250 and 375 kg ha-1 ) in field trials, and at month after last treatment after four weekly applications, the three higher rates significantly reduced root density and the two highest rates significantly reduced root length. He concluded that high rates of foliarly applied ferrous sulfate not only can lead to leaf blade phytotoxicity, but also causes phytotoxic effects on roots of Agrostis stolonifera, resulting in fewer and smaller roots.
Ferrous sulfate has previously been reported to significantly reduce dollar spot disease symptoms on Agrostis stolonifera at rates of 15.2 to 48.8 kg ha-1 (Reams, 2013; Hsiang, 2016; McCall et al., 2017). Rudland (2020) found that weekly applications of 25 kg ha-1 across 10 cultivars of Agrostis stolonifera, provided 74-78% disease suppression, whereas 125 kg ha-1 provided up to 99% disease suppression. Rudland (2020) found that weekly applications of 50 kg ha-1 had the highest level of control (81%), while minimizing phytotoxicity (0 to 2.5% depending on temperature). However, if frequent applications can be made, and darkening is not of concern, then a 3.5-day application interval at 25 kg ha-1 can provide 92% suppression with 2.5 to 5% phytotoxicity, depending on temperature (Rudland, 2020). However, for cooler periods, up to 125 kg ha-1 can be used to fully suppress dollar spot on Agrostis stolonifera.
Rudland (2020) found in tests where Clarireedia jacksonii was grown on agar amended with ferrous sulfate, the average EC50 (effective concentration to cause 50% inhibition of hyphal growth) value of 2.01 g L-1. If the ferrous sulfate were applied at a typical fungicide dilution rate of 1 L water per m2, then this is equivalent to 20 kg ha-1 or 200 g 100 m-2, which means that the spray application of ferrous sulfate has some inhibitory effect. In comparison, fungicides usually applied in dilutions at thousands of times of their EC50 values.
McCall et al. (2017) and Reams (2013) have recently hypothesized that iron from ferrous sulfate chelates with oxalic acid released by Clarireedia jacksonii, therefore limiting its pathogenicity. Venu et al. (2009) was the to point out that Clarireedia jacksoniiproduces oxalic acid, which causes wilting symptoms in the infected host plant leaf tissue (Walz et al., 2007). Rudland (2020) examined systemic activity of ferrous sulfate in the lab and in the field, and found that applications to roots did not change the resistance of leaves.
Recommendations for using ferrous sulfate against dollar spot
Iron sulfate heptahydrate is an inexpensive compound. For example, a 25 kg bag can be purchased at a fertilizer supplies store for less than U.S. $25, and hence, it costs around U.S. $25 per ha for a 25 kg ha-1 application rate to provide 75% disease suppression, and around US $125 for a 125 kg ha-1 application rate (up to 99% disease suppression). However, the higher rate gives greater discoloration and causes phytotoxicity especially during warmer times of the year (Rudland, 2020). Ferrous sulfate is a cost effective method of dollar spot control and can provide high levels of disease suppression, especially in the late Spring and early Fall at the higher rate, if darkening is not of great concern.
The darkening effect at significantly dissipates by seven days post treatment application and continues to decrease until there is little to no visible difference by three to four weeks post treatment application for rates of ferrous sulfate less than 50 kg ha-1. Rates greater than 50 kg ha-1, were observed to caused increasing levels of phytotoxicity during the hotter Summer months (July and August) but not during late Spring (May to June) and early Fall (September to November). Therefore, weekly application rates of ferrous sulfate up to 125 kg ha-1 can be used in the late Spring and early Fall to suppress dollar spot disease up to 99%, but we do not recommend anything over 50 kg ha-1 ferrous sulfate in the hotter Summer months to avoid phytotoxicity.
Some golf courses in southern Ontario, Canada have implemented turfgrass disease control programs using ferrous sulfate for years now. They use little or no fungicides on fairways, and on greens, only use fungicides when disease is severe (against dollar spot and snow mold diseases, and rarely for outbreaks of Pythium blight, brown patch or anthracnose). They apply ferrous sulphate at 25 kg ha-1 on 7 to 14 day intervals throughout the growing season, with additions of urea (0.3 kg ha-1 on 7 to 14 day intervals), wetting agents, magnesium compounds and nitrate compounds to address issues of nutrient deficiency associated with frequent use of ferrous sulphate.
요약
철(Fe)은 식물성장에 필요한 17대 필수원소 중 하나이며 유기체에 있어서 세포단위의 대사과정부터 관여하는 중요한 영양원소이다. 그러나 식물체 내의 과도한 수용성 철의 축적은 식물성장에 부정적인 영향을 줄 수 있는 유해한 수산기 라디칼이 형성의 원인이 되기도 한다. 황산철(Ferrous sulfate)은 잔디관리에 있어 잔디의 녹색도를 향상시키는 목적으로 오랫동안 사용이 되어왔으며, 최근에는 Microdochium patch 혹은 dollar spot과 같은 잔디병을 감소시키기 위한 목적으로 황산철의 사용에 대한 연구가 진행되고 있다. 본 연구에서는 황산철이 식물체내에서 dollar spot에 어떤 기작으로 작용하는 가에 대한 설명과 황산철을 사용하여 dollar spot 을 방제하기 위한 방법을 제안하고자 한다.