Introduction
Turfgrasses play a significant role in mitigating anthropogenic pollutants in urban and rural environments by sequestering carbon dioxide and reducing factors that contribute to energy consumption and climate change (Selhorst and Lal, 2012). Their extensive use in diverse landscapes underscores the need to understand their physiological responses to abiotic stressors, particularly salt stress�a growing concern as soil salinity increases globally due to shifting climate patterns and water management practices (Haynes et al., 2021). Research on the effect of salt stress mechanisms in turfgrasses is limited.
Salt stress impairs essential physiological functions, including photosynthesis, osmotic regulation, and nutrient transport (AcostaMotos et al., 2017). This leads to a measurable decline in turfgrass quality and growth, with symptoms such as chlorosis, leaf burn, and growth inhibition, which collectively affect the ornamental and functional value of turfgrasses in urban ecosystems (Liu et al., 2011).
Emerging research has highlighted the role of the ARGONAUTE2 (AGO2) gene in mediating responses to salt stress through RNA interference (RNAi) pathways (Ma et al., 2018). The AGO2 protein, a critical RNA-binding protein, is integral to posttranscriptional gene-silencing mechanisms that involve small RNAs, including microRNAs (miRNAs) and small interfering RNAs (siRNAs) (Tan et al., 2009). These molecules guide AGO2 to specific mRNA targets, allowing it to mediate stress-responsive gene silencing and modulate gene expression, which is essential for maintaining homeostasis under salt stress conditions (Biggar and Storey, 2015). In Schizosaccharomyces pombe, AGO1 loaded with siRNAs localizes to pericentromeric regions, where it facilitates H3K9 methylation by recruiting the H3K9 methyltransferase Clr4 (Yu, 2016). This mechanism illustrates how ARGONAUTE proteins can regulate histone modifications to control gene expression. Similarly, under abiotic stress conditions such as salt stress, plant AGO2 proteins can induce histone modifications, such as H3K9me2 (dimethylation) or H3K27me3 (trimethylation), to suppress gene expression. By increasing these methylation marks in the promoter regions of specific stress-responsive genes (Liu et al., 2018), AGO2 promotes gene silencing, thereby modulating the plant’s adaptive response to environmental stressors. The diverse functions of AGO proteins are reflected in their involvement in a wide range of biological processes. These include growth and development, stress responses, defense mechanisms, alternative splicing, and DNA repair (Fang and Qi, 2016). For example, in rice, ARGONAUTE2 has been reported to increase grain length and tolerance to salt stress via activation of Big GRAIN2 (BG3) (Yin et al., 2020).
ARGONAUTE2 (AGO2) gene is crucial for RNA silencing pathways and plays a significant role in plant stress responses (Liang et al., 2023). Extensive research has been published with a focus on revealing the adaptation mechanisms of zoysiagrasses to environmental stresses like salinity, drought, and low temperatures, with analyses concentrating on gene and transcript levels related to these stress responses (Wang et al., 2020). However, no studies have been reported on AGO2 in the Zoysia genus. Therefore, we conducted a comparative analysis to identify homologous AGO2 genes in Zoysia japonica, Zoysia sinica, Zoysia matrella, Zoysia pacifica, Zea mays, and Sorghum bicolor (Table 1). In this study, we used in-silico approaches to identify and characterize AGO2 genes in Zoysia japonica, Zoysia sinica, Zoysia matrella, Zoysia pacifica, Zea mays, and Sorghum bicolor. Zoysia japonica, Zoysia matrella, and Zoysia pacifica are major turfgrass species distributed in Korea, while Zoysia sinica is a representative species inhabiting coastal areas, making it particularly suitable for analyzing salt tolerance-related genes such as AGO2. Zea mays and Sorghum bicolor were selected to compare the AGO2 genes in the four Zoysia species with evolutionarily related species, providing insights into the evolutionary divergence of the AGO2 gene. Each species harbors a single AGO2 gene, which we analyzed in terms of gene structure, transcription regulatory elements, and protein structural predictions. This research advances the understanding of AGO2 gene regulation and salt stress response in four zoysiagrasses and related grasses, aiming to shed light on the genetic diversity within AGO2 that could be harnessed to enhance turfgrass resilience.
Table 1
List of ARGONAUTE2 genes predicted in the four Zoysia species, Zea mays, Sorghum bicolor and their sequence information.
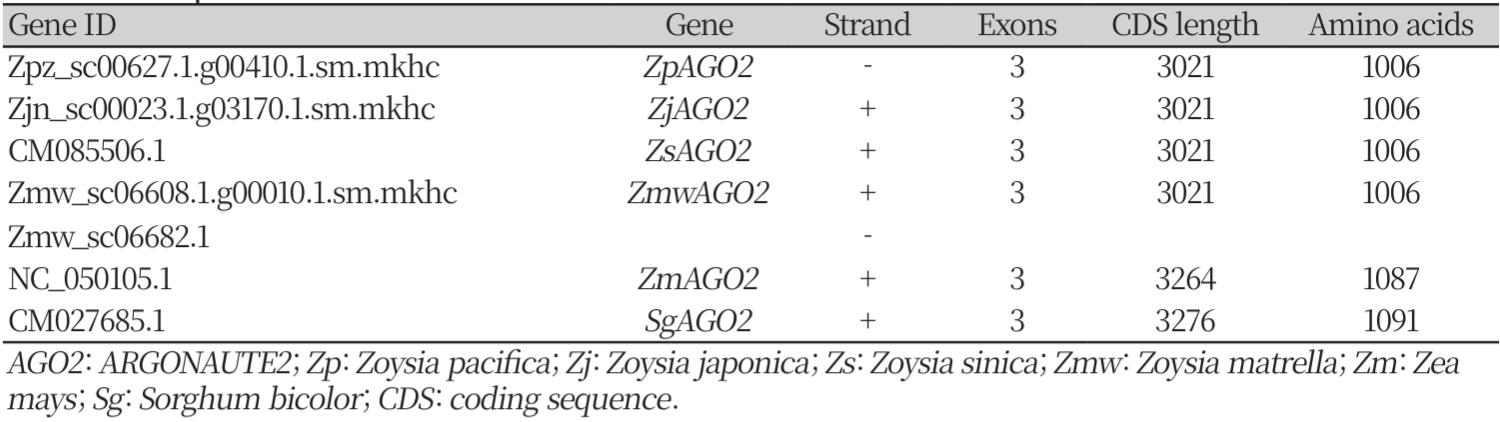
Materials and methods
Identification and sequence data retrieval of Zoysia ARGONAUTE2 (AGO2) genes
The full coding sequence and genomic DNA sequences of the AGO2 gene from Arabidopsis thaliana (AtAGO2), Zea mays (ZmAGO2), Sorghum bicolor (SgAGO2) were obtained from the ʻThe National Center for Biotechnology Information’ (https:// www.ncbi.nlm.nih.gov/). The genomic sequences of Zoysia japonica (Zj), Zoysia matrella (Zmw), and Zoysia pacifica (Zp) were obtained from the ʻZoysia Genome Database’ (http://zoysia.kazusa.or.jp/).
To identify AGO2 genes Zoysia japonica, Zoysia matrella and Zoysia pacifica, a BLASTP search using the AtAGO2 protein sequence as a query was conducted. The sequence of putative AGO2 gene in Zoysia sinica was obtained from the whole genome sequencing data in our lab (unpublished data). After retrieving the BLASTP results, the NCBI Conserved Domain database was used to search for conserved domains. The intron-exon structure of the predicted AGO2 genes was constructed by manually aligning the coding sequences (CDS) with the corresponding genomic sequences.
The phylogenetic tree was anaylzed with Molecular Evolutionary Genetics Analysis (MEGA) 11, using maximum likelihood method and JTT matrix-based model (Jones et al., 1992) with 100 bootstrap replications (Tamura et al., 2021).
Identification of Zoysia AGO2 upstream regulatory region (URR)
A 2 kb sequence upstream of the transcription start site was extracted to identify the upstream regulatory domains of the predicted AGO2 genes. Transcription Start Sites Plant (TSSP) was used to estimate the probable promoter and TATA box sites (Shahmuradov et al., 2017). The transcription binding sites in the URR were predicted using PlantTFDB (Jin et al., 2016).
Protein structure prediction
The physical and chemical parameters of the predicted AGO2 proteins in the Zoysia grasses was computed using the ProtParam tool from Expasy (Gasteiger et al., 2005). Using SWISS-MODEL, homology modelling, the tertiary structures of the AGO2 proteins were predicted and Chimera 1.15 was used for visualization of the protein structures (Kelley et al., 2015; Kiefer et al., 2009). After retrieving all the BLAST results, the NCBI Conserved Domain database was used to conduct a search for conserved domains.
Results and Discussion
Identification and characterization of the AGO2 genes in the four zoysiagrasses and Zea mays, Sorghum bicolor
The sequence of Zoysia matrella AGO2 gene, downloaded from the Zoysia genome database was found to be incomplete. Upon performing protein sequence alignment between Zoysia matrella and other Zoysia species, we identified that the CDS and the gene’s latter portion were identical to Zoysia japonica, allowing us to recover the missing upstream section.
Each predicted AGO2 gene consisted of three exons and the coding sequence (CDS) lengths and amino acid counts varied across species. In Zoysia japonica (ZjAGO2), Zoysia pacifica (ZpAGO2), Zoysia sinica (ZsAGO2), and Zoysia matrella (ZmwAGO2), the CDS length was 3,021 bases, each encoding a protein of 1,006 amino acids. In Zea mays (ZmAGO2), the CDS length was longer, at 3,264 bases, encoding a protein with 1,087 amino acids and Sorghum bicolor (SgAGO2) had a CDS length of 3,276 bases, encoding a protein of 1,091 amino acids.
All analyzed species, contained Duf, ArgoN, ArgoL1, PAZ, and Piwi-like superfamily domains in their gene structure (Fig. 1). These conserved domains are integral to plant responses under abiotic stress conditions, particularly salt stress (Martín-Merchán et al., 2023), as they play essential roles in RNA interference (RNAi) pathways that regulate gene expression and stabilize cellular processes during stress.
Fig. 1
Phylogenetic relationship of AGO2 genes among the four Zoysia species, Zea mays and Sorghum bicolor using maximum likelihood method with 100 bootstrap replications. The purple box represents the Duf domain, the orange box represents the Argon domain, the green box represents the ArgoL1 domain, the yellow box represents the PAZ domain, the blue box represents the Piwi-like superfamily domain. The black boxes represent exons and dotted lines represent introns. A and C denotes major groups, and B denotes subgroups. AGO2: ARGONAUTE2; Zp: Zoysia pacifica; Zj: Zoysia japonica; Zs: Zoysia sinica; Zmw: Zoysia matrella; Zm: Zea mays; Sg: Sorghum bicolor.
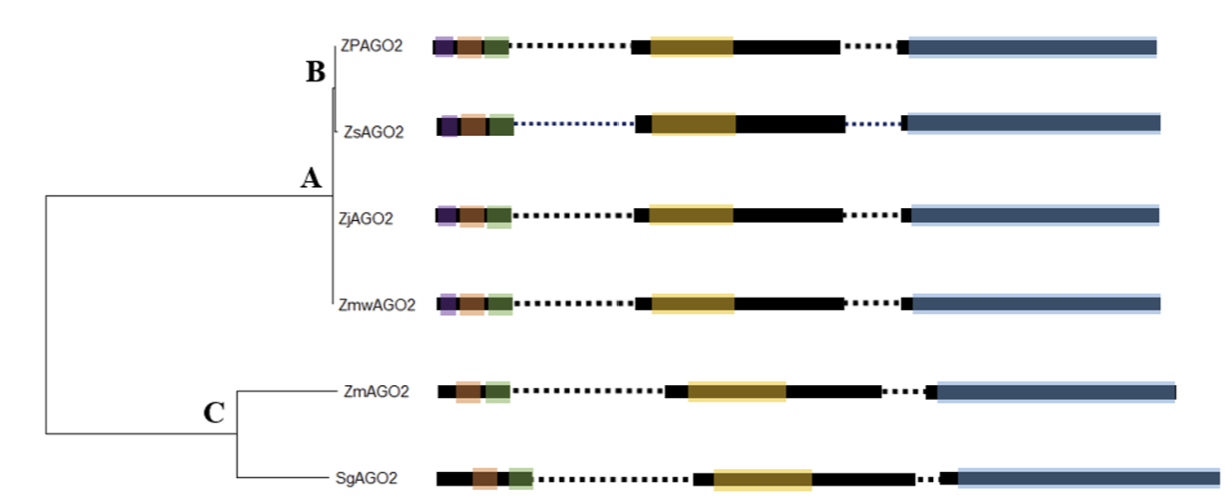
The ArgoN and ArgoL1 domains contribute to the structural stability necessary for binding small RNAs, which are central to silencing stress-inducible genes and modulating plant responses under saline conditions (Martín-Merchán et al., 2023).
The PAZ domain is critical for the recognition and binding of small RNA molecules, aiding in enhancing salt tolerance by regulating stress-responsive gene expression through RNA silencing pathways (Li et al., 2024). The Piwi-like superfamily domain is essential for gene regulation processes that are necessary for coping with salt stress. This domain facilitates interactions within the RNA-induced silencing complex (RISC), which downregulates target genes that could otherwise interfere with the plant's stress tolerance mechanisms (Qian et al., 2011).
Despite not being essential for plant survival, research has shown that the DUF family plays a crucial role in physiological processes, including plant growth, development, and responses to biotic and abiotic stresses. For example, DUF family genes have been suggested to contribute to cell protection and repair mechanisms under salt stress conditions in Oryza sativa (Ma et al., 2024). Notably, the presence of the DUF domain exclusively in Zoysia species compared to Zea mays and Sorghum bicolor, may contribute to its enhanced salt tolerance.
Zoysia AGO2 upstream regulatory region analysis and identification of transcription factor binding sites
Transcription binding sites (TFs) play a crucial role in regulating gene expression by selectively activating or repressing the transcription of target genes through interactions with upstream regulatory regions (URRs) (Parisutham et al., 2022). In this study, a 2 kb sequence upstream of the transcription start site was extracted for promoter and TATA box predictions, revealing that promoter positions were highly conserved among almost all Zoysia species analyzed. However, we were unable to identify promoter and TATA box sequences in Zoysia sinica. This may suggest differences in its regulatory mechanisms, where Zoysia sinica might utilize alternative regulatory elements, such as GC-rich regions or transcription factor binding sites, within its promoter region (Bernard et al., 2010). These variations could be an evolutionary adaptation to specific environmental conditions. The conservation of promoter elements across Zoysia species may indicate a shared regulatory mechanism for gene expression in response to environmental stressors, with Zoysia sinica potentially differing in its regulatory architecture. (Fig. 2.)
Fig. 2
Analysis of the upstream regulatory region of the AGO2 genes predicted in the four Zoysia species, Zea mays and Sorghum bicolor. The predicted promoter and TATA box sites are denoted in green and yellow, respectively. The transcription start site (+1) is marked by a red triangle. Predicted enhancer sites are marked in blue. AGO2: ARGONAUTE2; Zp: Zoysia pacifica; Zj: Zoysia japonica; Zs: Zoysia sinica; Zmw: Zoysia matrella; Zm: Zea mays; Sg: Sorghum bicolor.
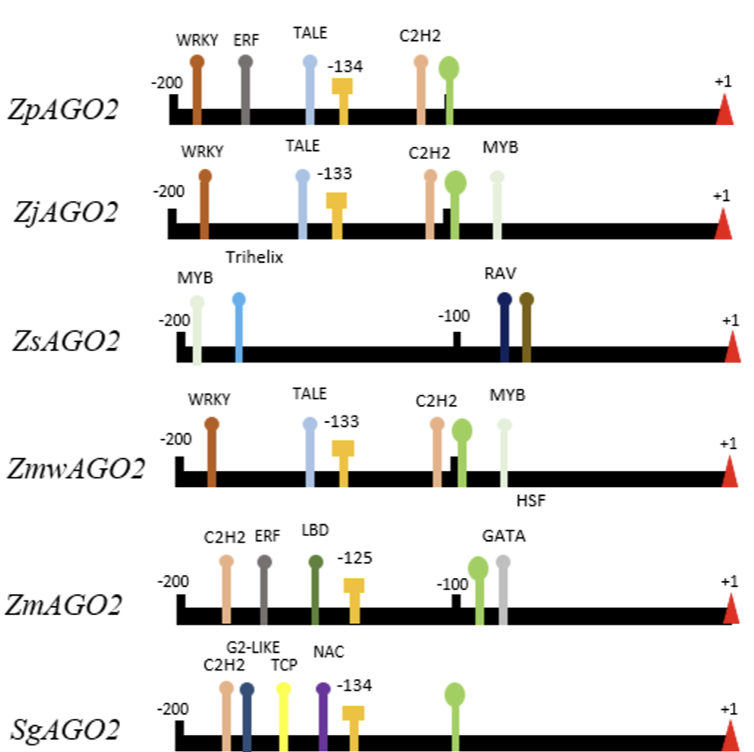
The analysis of TF binding sites within these URRs identified binding motifs from various transcription factor families, including ARF, B3, BBR-BPC, bZIP, CPP, C2H2, ERF, HD-ZIP, MIKC_MADS, MYB, TALE, TCP, Trihelix, G2-Like, NAC, SBP, WRKY, WOX, YABBY, and ZF-HD (Table 2). Notably, TF families known for salt stress involvement include NAC (Guo and Gan, 2006), WRKY (Jiang et al., 2017), bZIP (Noh et al., 2021), and MYB (Yang et al., 2012).
Table 2
Transcription factor binding motifs identified in the upstream regulatory regions of AGO2 Genes in four Zoysia species, Zea mays, and Sorghum bicolor.
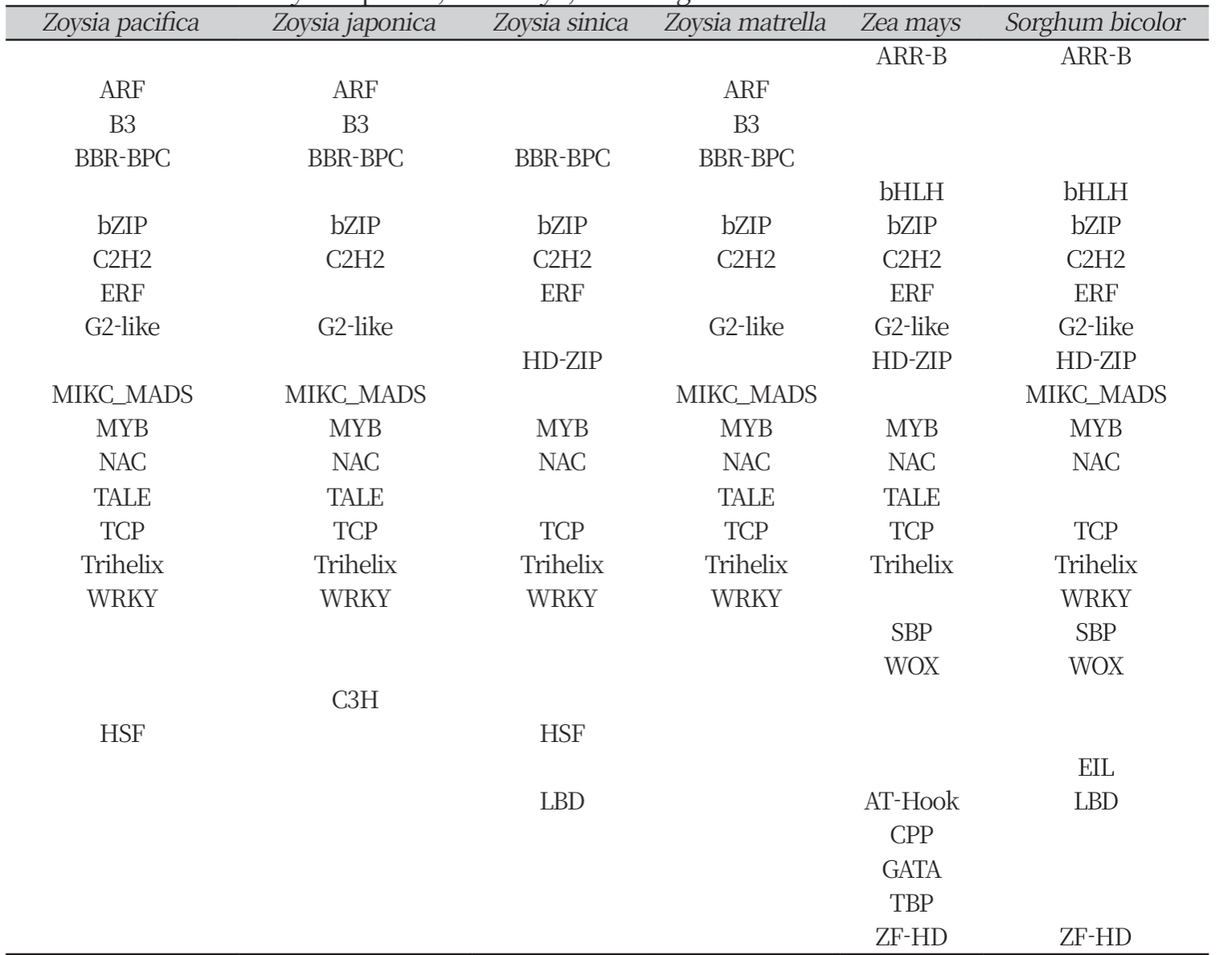
The NAC transcription factor family (NAM, ATAF, CUC), one of the largest plant TF families, is associated with stress response (Yuan et al., 2019). In our study, NAC motifs were observed in six species’ AGO2 genes. The upregulation of NAC genes has been linked to increased stress resilience, supporting plant survival and development under high-salinity conditions in Oryza sativa (Sun et al., 2022).
MYB and MYB-related motifs were found in the URRs of all analyzed AGO2 genes in Zoysia species, Zea mays, and Sorghum bicolor. MYB transcription factors are commonly associated with salt stress adaptation, as well as leaf senescence and nitrogen remobilization in Arabidopsis, Oryza sativa. (Cao et al., 2023). These TFs contribute to physiological adjustments that enhance tolerance to saline environments.
Also, bZIP transcription factor family (basic leucine zipper) is one of the key transcription factor groups in plants and is closely associated with stress responses in Arabidopsis (Jakoby et al., 2002). In this study, bZIP motifs were observed in the URRs of AGO2 for all entries investigated.
The bZIP transcription factors play a critical role in responding to salt and drought stress by contributing to osmotic regulation, reactive oxygen species (ROS) detoxification, and the regulation of stress-induced gene expression (Obertello et al., 2010). Additionally, bZIP transcription factors are involved in both ABA (abscisic acid)-dependent and ABA-independent pathways within signaling networks, enabling plants to adapt to extreme environmental conditions (Noh et al., 2021).
Furthermore, WRKY TF binding motifs were present in AGO2 of five entries except Zea mays. The WRKY gene family is integral to the salt stress response, with several WRKY-regulated pathways well-documented in Arabidopsis (Chen et al., 2010).
The TF binding sites belonging to this family influences stress tolerance by modulating stress-induced gene expression, improving cellular stability under salt stress conditions. The presence of key salt stress-related TF binding sites in the regulatory regions of AGO2 genes across all of Zoysia grasses, Zea mays, and Sorghum bicolor suggest that these TF binding sites may facilitate adaptive responses in AGO2 genes under salt stress, positioning them as potential targets for enhancing stress resilience in these turfgrass species.
AGRONAUTE2 protein sequence analysis and structure prediction
The single amino acid polymorphism (SAP) analysis of the conserved domain in the predicted ARGONAUTE2 proteins revealed that, at position 113bp in Duf domain, other Zoysia species had valine (V), while Zoysia sinica uniquely had alanine (A). At position 426bp in Paz domain, other zoysia species had tryptophan (W), while Zoysia sinica uniquely had isoleucine (I). Additionally, at position 631bp in Piwi-like superfamily domain, Zoysia japonica and Zoysia matrella possessed serine (S), whereas Zoysia pacifica and Zoysia sinica had alanine (A) (Fig. 3).
When comparing Zoysia species to Zea mays and Sorghum bicolor, In the Piwi-like superfamily domain of Zea mays and Sorghum bicolor, an insertion of glutamic acid (E), proline (P) was observed at position 601bp, while a deletion of proline (P), serine (S) occurred at position 571bp. Notably, in Sorghum bicolor, an additional insertion of glycine (G) was identified at position 741bp, whereas Zea mays exhibited a deletion of proline (P), serine (S), serine (S) at position 988bp (Fig. 3). Some research suggests that AGO2 proteins may undergo structural modifications under stress conditions, potentially influencing their functional activities (Zhang et al., 2011). This suggests that AGO2 may function as a regulatory protein involved in gene expression control rather than acting strictly as an enzyme. The conservation of AGO2 sequences across diverse plant species supports its essential role in environmental adaptation and stress response. These variations suggest that species can be classified into two major groups: one consisting of Zoysia species and the other including Zea mays and Sorghum bicolor. Within the Zoysia group, Zoysia pacifica and Zoysia sinica form a subgroup, indicating further divergence among the Zoysia species (Fig. 1).
The physical and chemical properties of the predicted AGO2 proteins in Zoysia and related grasses were analyzed. The properties of ZpAGO2, ZsAGO2, ZmwAGO2 and ZjAGO2 in Zoysia pacifica, Zoysia sinica, Zoysia matrella and Zoysia japonica, respectively, were nearly identical, both containing 1,006 amino acids, with a molecular weight of approximately 110,250 Da and an isoelectric point (PI) of 9.35. In contrast, Zea mays (ZmAGO2) and Sorghum bicolor (SgAGO2) AGO2 proteins exhibited molecular weights of 109,988.64 Da and 116,481.88 Da, respectively, with slightly higher PI values of 9.44 and 9.41, indicating structural variations (Fig. 4). Additionally, secondary structure prediction of AGO2 in was conducted. Zoysia pacifica exhibited the highest proportion of alpha helices (24.21%) and beta sheets (21.00%). Zoysia japonica and Zoysia matrella displayed similar profiles with 24.02% alpha helices and 21.06% beta sheets. Zoysia sinica, with 23.96% alpha helices and 21.17% beta sheets. Zea mays exhibited 24.03% alpha helices and 20.84% beta sheets, Sorghum bicolor had 23.97% alpha helices and the lowest beta sheet content (20.53%).
Based on the SAP analysis, in Zoysia sinica, we observed a substitution where tryptophan (W) was replaced by isoleucine (I) at position 426bp in the Piwi-like superfamily domain, in compared to Zoysia matrella, Zoysia pacifica and Zoysia japonica. In Zoysia pacifica and Zoysia sinica, serine (S) was replaced by alanine (A) at position 631bp in the Piwi-like superfamily domain, differs from that of Zoysia matrella and Zoysia japonica (Fig. 3). However, these SAP changes did not affect the structure of the AGO2 protein structure; given the varying salt tolerance observed among Zoysia species, they are likely related to salt stress adaptation. Therefore, further research is needed in the future to fully understand their role.
Fig. 3
Multiple sequence alignment of the predicted AGO2 proteins. The colors correspond to the amino acid identity and the bar graph indicates the degree of conservation between the sequences. The predicted Duf domain is indicated by the gray box above the sequence, ArgoN domain by the red box, the ArgoL1 domain by the yellow box, the PAZ domain by the green box, the Piwiago-like domain by the blue bar, SAP by the purple bar.
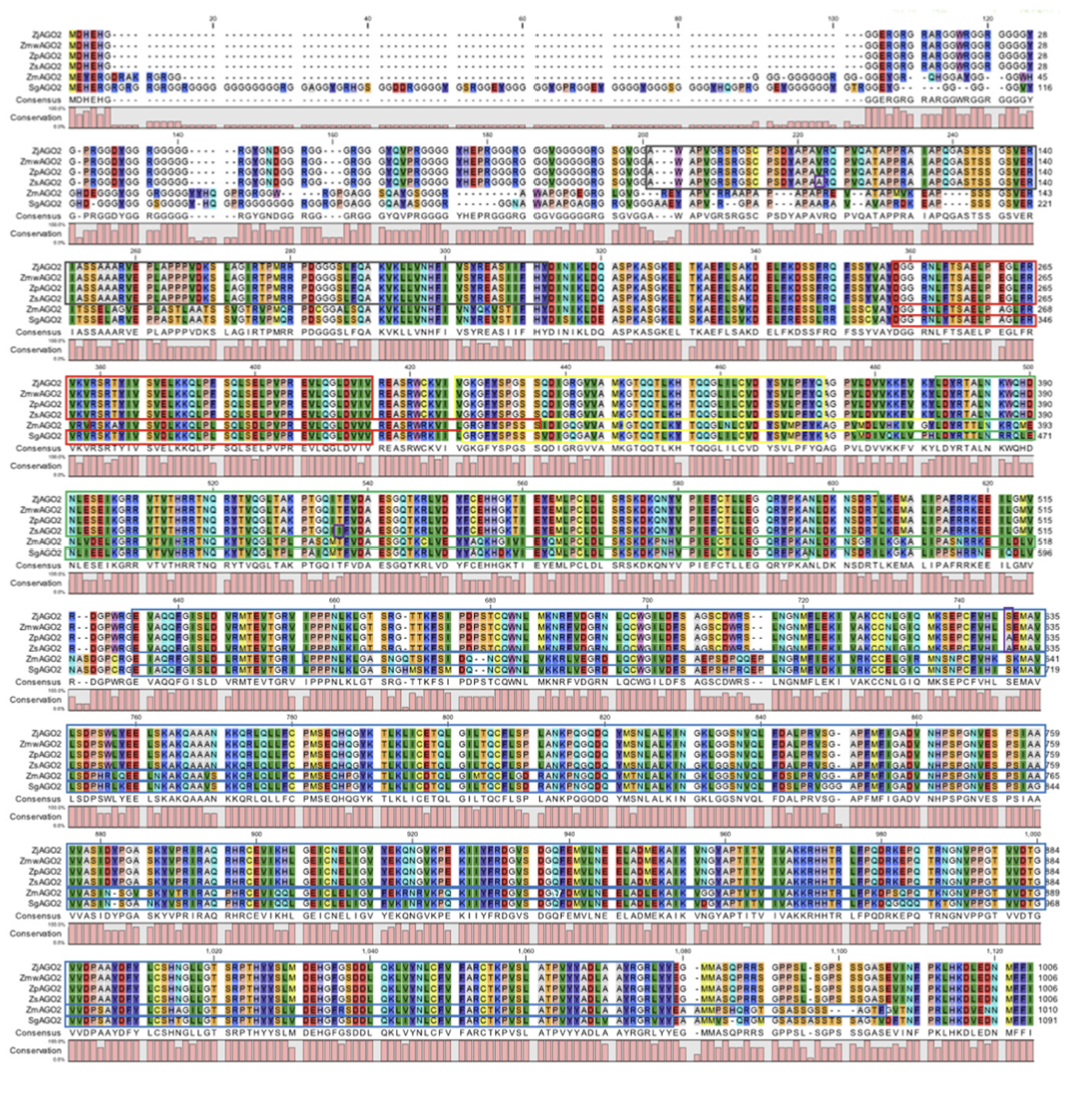
Fig. 4
Four Zoysia species, Zea mays and Sorghum bicolor AGO2 proteins physical, chemical, and structural analysis. For each AGO2 protein, the tertiary structure was predicted using PHYRE2 (image colored by rainbow N to C terminus). AGO2: ARGONAUTE2; Zp: Zoysia pacifica; Zj: Zoysia japonica; Zs: Zoysia sinica; Zmw: Zoysia matrella; Zm: Zea mays; Sg: Sorghum bicolor.
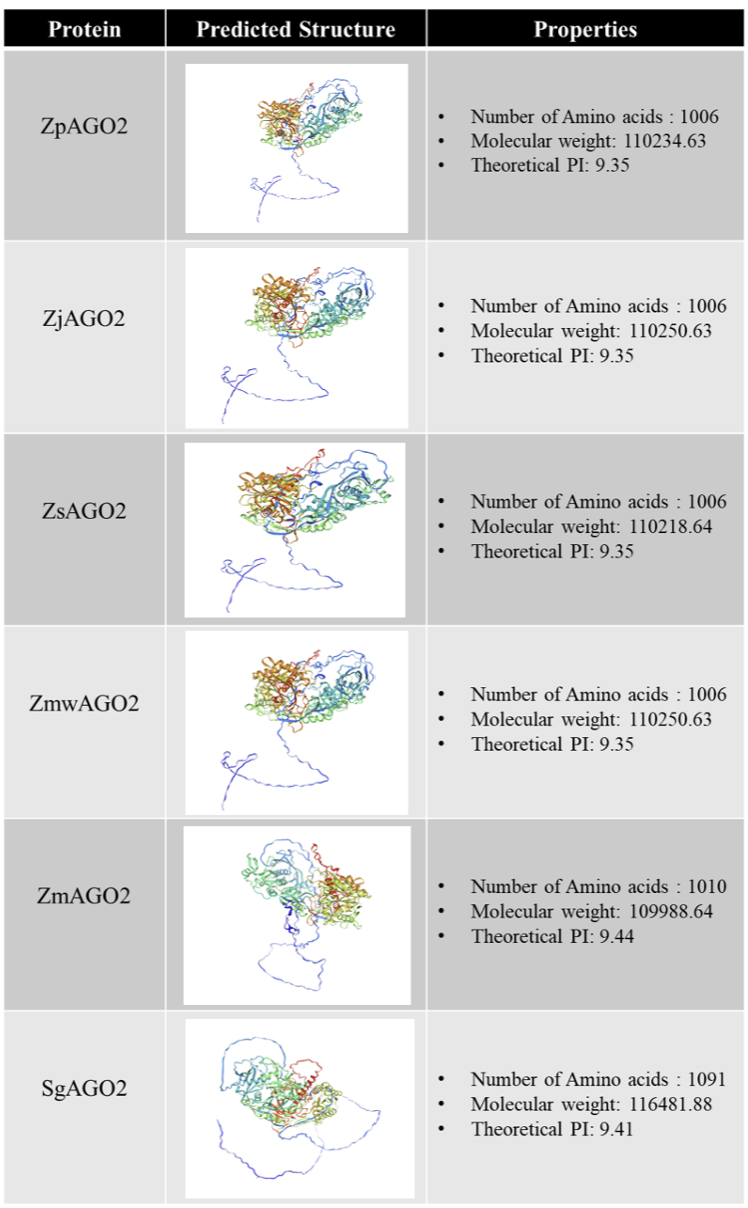
Conclusion
The accelerating impact of climate change on biological systems is imposing greater abiotic stress on plants, including increased temperatures, irregular precipitation, and heightened soil salinity (Ullah et al., 2021). While much research has focused on primary agricultural crops, relatively few studies have addressed these challenges in turfgrasses, which play a crucial role in ecosystem services and urban landscaping.
In this study, we characterized the AGO2 gene in four Zoysia species along with homologous genes in Zea mays and Sorghum bicolor, focusing on their roles in salt stress adaptation. Our findings highlight the genetic variations in AGO2 proteins across these species, which may contribute to their differential responses to salt stress. We identified conserved domains and unique TF binding sites and predicted the protein structures, elucidating potential regulatory mechanisms of AGO2. Additionally, based on the analysis results of the four Zoysia species, and consistent with sequence comparisons in our studies, AGO2 proteins are shown to be highly conserved. The results of this research expand the limited knowledge base on stress tolerance in zoysiagrasses and indicate that AGO2-mediated pathways could be utilized to enhance salt tolerance in turfgrass species.
Significantly, this study represents the first investigation of the salt stress-related gene AGO2 in Zoysia sinica, marking an important milestone in understanding the stress adaptation mechanisms of this species. With the rapid increase in soil salinization caused by recent climate change, there is a growing demand for salt-tolerant plants (Mukhopadhyay et al., 2021). This study identifies the genetic mechanisms underlying salt tolerance in Zoysia sinica, highlighting their potential for use as climate-adaptive turfgrasses or plants for environmental restoration.
The in-silico analysis revealed that AGO2 exhibited similar genetic patterns across the four Zoysia species, and no clear correlation with differences in salt tolerance observed in field conditions was identified. To confirm these findings and explore their practical application, further studies involving the functional validation of AGO2 gene expression under salt stress, accompanied by field trials, would be essential. By deepening our understanding of AGO2 role in salt stress response, this research ultimately supports the sustainable development of resilient turfgrass varieties, providing a foundation for climate-adaptive turfgrass breeding and management practices.
Acknowledgment
This research was funded by Agricultural New Technology Industry-Academia Cooperation Support Project (2024-BA-02-005) of the Korea Agriculture Technology Promotion Agency (KOAT).
Authors Information
Seo-young Kim, https://orcid.org/0009-0007-7575-4842
Chetan Kaur, https://orcid.org/0000-0001-6226-7921
Young-Sun Kim, https://orcid.org/0000-0002-5645-7021
Donghwan Shim, https://orcid.org/0000-0002-0223-595X
Eun-Ji Bae, https://orcid.org/0000-0003-4597-8873
Geung-Joo Lee, https://orcid.org/0000-0002-3774-1860